The climate of the Antarctic and its variability
- This page is part of the topic The Antarctic environment in the global system
In the summer, when the sun is above the horizon for long periods, the Antarctic receives more solar radiation than the tropics, but the highly reflective ice- and snow-covered surfaces reflect much of this radiation back to space, aided by a relatively cloud-free atmosphere that contains little water vapour. This reflection is one of the important feedback mechanisms found in the ice-covered polar regions, because it enhances cooling. Where snow melts, exposing large patches of bare (dark) ground, or where sea ice melts exposing ice free (dark) ocean, solar radiation will be absorbed rather than reflected, and the environment will warm.
The Polar Plateau of East Antarctica experiences very low temperatures because of its high elevation, the lack of cloud and water vapour in the atmosphere, and the isolation of the region from the relatively warm maritime air masses found over the Southern Ocean. The very cold temperatures in the interior of Antarctica year-round, coupled with its isolation from warm, moist air masses, mean that precipitation there is very low, with only about 5 cm water equivalent falling per year (King and Turner, 1997[1]). That makes much of Antarctica a desert and the driest continent on Earth. The low temperatures mean that there is very little evaporation and sublimation, so that although the amount of precipitation is small, it builds up year by year to form the ice sheet. Many blizzards tend to be fallen snow resuspended, rather than new snow.
Temperatures are much less extreme in the Antarctic coastal region than on the plateau. In general, at most of the coastal stations the monthly mean summer temperatures never rise above freezing point, although daily temperatures may show excursions above freezing in places during summer. There are exceptions. The highest temperatures on the continent are found on the western side of the Antarctic Peninsula where there is a prevailing northwesterly wind; there temperatures can rise to several degrees above freezing during the summer, and monthly means are positive for 2-4 months of the year. Temperatures also tend to be above freezing at times in places like the Schirmacher Oasis near the coast in Dronning Maud Land, where there are ice-free lakes in the austral summer.
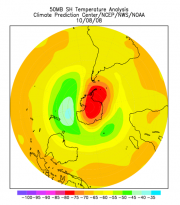
Because of the lack of incoming solar radiation, the Antarctic stratosphere in winter is extremely cold. A strong temperature gradient develops between the continent and mid-latitudes (Figure 1.13), isolating a pool of very cold air above Antarctica. Very strong winds develop along this thermal gradient. They are stronger than the equivalent winds found in the Arctic, because the Equator-to-pole temperature difference is larger in the south. The pool of cold air and its strong surrounding winds together form the polar vortex. It plays an important part in determining the atmospheric circulation of the high southern latitudes, as well as in the formation of the ozone hole, where the polar vortex acts as a ‘containment vessel’ allowing chlorofluorocarbon compounds (CFCs) to build up during the winter.
The Antarctic climate system varies on time scales from the sub-annual to the millennial and is closely coupled to other parts of the global climate system. On the longest time scales it fluctuates on Milankovitch frequencies (20 ka, 41 ka, 100 ka) in response to variations in the Earth’s orbit around the sun that cause regular variations in the Earth’s climate on these recurrent time scales. We discuss the variability of the Antarctic climate system on these longer timescales in Antarctic climate and environment history in the pre-instrumental period.
Proxy data from ice cores show that since the Last Glacial Maximum (LGM) at about 21 ka before present (BP) there have been a number of climatic fluctuations across the continent. One of the most marked was the Mid Holocene warm period, which is present in various records from Antarctica. Ice cores also reveal shifts in the intensity of the atmospheric circulation, with the westerlies weakening at 5,200-5,400 years ago and strengthening around 1,200 years ago.
Because long time-series of observations did not begin until the IGY (1957-58), the instrumental period in Antarctic is only about 50 years long (measurements started before then but were not continuous). Since proxy data show oscillations on longer time scales than 50 years, it is accepted that the instrumental period only provides a snapshot of change in the Antarctic. Nevertheless, it shows the complexity of change and a mix of natural climate variability and anthropogenic influence, as discussed in Observations, data accuracy and tools and Antarctic climate and environment change in the instrumental period.
Reliable weather charts for high southern latitudes have only been available since the late 1970s. They reveal a great deal about the patterns in the atmospheric circulation of high southern latitudes. The most pronounced climate variability over this period is evident in changes in the SAM. Over the last few decades there has been a marked drop in pressure around the Antarctic coast and an increase in mid-latitudes. The increase in the pressure gradient across the Southern Ocean has strengthened the surface winds, which in turn has affected ocean currents and the distribution of sea ice. Studies of coupled ocean-atmosphere general circulation models show that the strength of the SAM should increase as the Earth warms, confirming what is known from observations.
But the strength of the SAM is also modulated by the ozone hole over Antarctica. Stratospheric ozone is an important constituent of the upper atmosphere above the Antarctic, where ozone levels began to decline in the late 1970s following widespread releases of CFCs and halons into the atmosphere. We now know that the presence of CFCs in the Antarctic stratosphere results in a complex chemical reaction during the spring that destroys virtually all ozone at altitudes between 14 and 22 km, especially within the polar vortex where temperatures are coldest. The depletion in ozone maintains the cooling within the polar vortex, which accentuates the winds around the vortex. This strengthening of the winds at high altitude in spring then propagates downwards in the atmosphere through time, strengthening surface winds during the summer and autumn. Thus one effect of the ozone hole has been to accentuate the SAM signal, further strengthening the westerly winds around the Antarctic during the summer and autumn.
In the strengthened SAM, with its stronger winds, we see two examples of changes from outside the region having a profound impact on the Antarctic environment. One impact comes from the CFCs responsible for the ‘ozone hole’, and the other comes from greenhouse gas emissions responsible for global warming. Both CFCs and greenhouse gases have mainly been released in the Northern Hemisphere during the industrial era. Both have had, and continue to have, a profound effect on the radiation balance of the Antarctic atmosphere.
Although the Antarctic is far removed from where most of the solar energy enters the system at tropical latitudes, it is still influenced by variability in tropical conditions, and signals of low latitude climate variability can be identified in Antarctica and the Southern Ocean (Turner, 2004[2]). In addition, there is increasing evidence that signals can also be transmitted in the opposite direction from high to low latitudes.
Statistically significant long-range linkages between high and low latitudes go by the name of teleconnections. They can take place via the atmosphere and/or the ocean, although the timescales are usually rather different. The most rapid teleconnections generally occur via the atmosphere, with storm track changes occurring on the scale of days or weeks. The El Niño-Southern Oscillation (ENSO) is one of the largest climatic cycles on Earth, functioning on the scale of years to decades. It has its origins in the tropical Pacific Ocean, but its effects can be felt across the world. As discussed in a number of places in this volume, ENSO signals can be identified in the physical and biological environment of the Antarctic, although some of the links are not robust and there can be large differences in the extra-tropical response to near-identical events in the tropics.
The circulation of the upper layers of the ocean can change over months to years, but the deep ocean and the global thermohaline circulation (THC) require decades to centuries to respond. At the other extreme, fast wave propagation in the ocean takes place on timescales of just a few days.
About 30 years of reliable atmospheric reanalysis outputs are available for the high southern latitudes, so it has been possible to establish the nature of the broad-scale teleconnections typical of the Southern Hemisphere. There is evidence of decadal timescale variability in some of these linkages, but with such a short data set it is not possible at present to gain insight into how the teleconnections may vary on longer timescales. High resolution ice core records collected from areas where the rate of precipitation of snow is high shed some light on teleconnections over the century timescale, and where the rate of accumulation is high enough they can even give seasonal data, which is important since some teleconnections are only present in individual seasons.
Links between the climates of the northern and southern hemispheres can be found, but they vary with time as discussed in The last million years and Holocene climate changes. Through most of the Holocene (roughly the past 12,000 years) northern hemisphere events have lagged behind southern hemisphere ones by several hundred years. That has changed in recent decades, and the northern hemisphere signal of rising temperature since about 1850 AD has paralleled the southern hemisphere one. Temperature change in the two hemispheres now appears to be synchronous - a radical departure from former times, which suggests a new and different forcing, most likely related to anthropogenic activity in the form of enhanced greenhouse gases. Comparing data on winds and temperatures from northern and southern hemisphere ice cores confirms that the wind/temperature fields of today differ from those of the recent past indicating that the modern day atmosphere is not an analogue for that of the so-called Medieval Warm Period.
Changes associated with the changing climate are now plainly evident in the Antarctic, and will be discussed in detail in both the pre-instrumental and instrumental periods. They range from the glaringly obvious, like the collapse of the Larsen B ice shelf in February-March 2002, and the warming of various parts of the Antarctic Peninsula over the past 50 years (King, 1994[3]), to the rather more subtle, like the 0.2ºC warming of the Southern Ocean, which - though a small amount - represents a major transfer of heat when summed over a vast area.
References
- ↑ King, J.C. and Turner, J. 1997. Antarctic meteorology and climatology, Cambridge University Press, Cambridge, UK, 409 pp.
- ↑ Turner, J. 2004. The El Niño-Southern Oscillation and Antarctica, International Journal of climatology, 24, 1-31.
- ↑ King, J.C. 1994. Recent variability in the Antarctic Peninsula, International Journal of Climatology, 14(4), 357-369.