Antarctic climate and environment history in the pre-instrumental period
Chapter Editor: Dominic A. Hodgson
Authors: Nerile Abram, John Anderson, Luca Bargelloni, Peter Barrett, Michael J. Bentley, Nancy A.N. Bertler, Steven Chown, Andrew Clarke, Peter Convey, Alistair Crame, Xavier Crosta, Mark Curran, Guido di Prisco, Jane E. Francis, Ian Goodwin, Julian Gutt, Dominic A. Hodgson, Guillaume Massé, Valérie Masson-Delmotte, Paul A. Mayewski, Robert Mulvaney, Lloyd Peck, Hans-Otto Pörtner, Regine Röthlisberger, Mark I. Stevens, Colin P. Summerhayes, Tas van Ommen, Cinzia Verde, Elie Verleyen, Wim Vyverman, Christian Wiencke, Lorenzo Zane.
Introduction
This chapter reviews Antarctic climate and environmental history from deep time through to the early part of the instrumental period. First, the large scale changes that have shaped the climate and environments of Antarctica and its surrounding ocean are described; from its early origins as the centrepiece of the Gondwana supercontinent, though its subsequent breakup and isolation by the circumpolar Southern Ocean c. 180 Ma (million years) ago. This is followed by a description of the formation of the first ice sheets on the continent c. 34 Ma, and later, the development of the 100 ka (thousand years) glacial cycles that have characterised the last 0.9 Ma. The climate at the end of the last major glaciation, retreat of the ice sheet and its effects on global sea level, and the changing distribution of sea ice and its effect on climate are then described.
Our present interglacial, the Holocene is described in detail from a combination of ice cores, marine sediments, lake sediments and terrestrial records with a focus on both regional and continent-wide climate and environmental variability. This provides a background, against which the climate changes in the instrumental period (Chapter 4) and the predicted climate changes of the future (Chapter 5) can be compared. In order to facilitate such comparison, towards the end of the chapter we examine the evolutionary and biogeographical factors that have shaped the contemporary biota of the continent, including some of the physiological adaptations in the marine realm that have led to the distinctive marine fauna seen today. This section also highlights some of the reorganisations in species distributions that have occurred during the relatively minor natural climate changes of the Holocene that are being used to inform our understanding of the climate and biological changes that might be anticipated in the near future (Chapter 5).
The modern climate over Antarctica and the Southern Ocean results from the interactions within the ice sheet – ocean – sea ice – atmosphere system. Knowledge of how this system responds to past and present climate forcing and the phasing of climate events on regional to hemispheric scales is essential for understanding the behaviour of the Earth’s past and future climate. Studying the history of Antarctic climate and environment is also important as it provides the context for understanding present day climate and environmental changes. Together, climate and environmental studies allow researchers to determine the processes that led to the development of our present interglacial state, and to define the ranges of natural climate and environmental variability on timescales from decades to millennia that have prevailed over millions of years in the past. Once this natural variability is known we can more confidently determine when changes have exceeded the natural state. We can also study, for example, past warm periods to identify and understand the processes that might have caused them.
In Antarctica, past climates and environments can be reconstructed from a range of palaeoenvironmental records. Each of these provides different, yet often complementary, information on the patterns and mechanisms of change and they span a range of different timescales. Instrumental data from satellites, ground-based instruments and oceanographic surveys provide detailed information about past climates for much of the last five decades. However, for records that pre-date this ‘instrumental period’ it is necessary to use the stratigraphic records contained in lake sediments, marine sediments and ice cores, and for the longest time periods, geological records on land and in deep ocean sediments.
Geological records are used to study the distant past, or ‘deep time’. A particular value of studying climate in the distant past is that we need to go back more than 30 million years to find atmospheric CO2 concentrations at more than twice pre-industrial levels, which we are projected to encounter by the end of this century. Geologists have termed this the “greenhouse” world, and both the biota and geothermometers indicate it was indeed much warmer, in contrast to the “icehouse” world that was initiated with the first ice sheets on Antarctica 34 million years ago. In the pages that follow we will briefly outline the main features of this world and chart the changes that have taken place as CO2 levels declined and persistent ice sheets grew in the Antarctic.
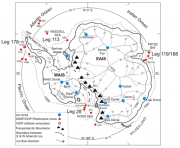
Geological records of past climate on land in Antarctica are generally accessible only in mountains around the margin or in the Transantarctic Mountains due to the thick continental ice cover (Figure 3.1). These records either largely predate Gondwana breakup, which began around 180 million years ago, or in the case of sediments deposited since glaciation began they are mostly patchy and difficult to date (Barrett, 1996[2]). However a few places, like the Antarctic Peninsula, have well-exposed post-breakup sedimentary strata that predate the first ice sheets. James Ross Island and Seymour Island in particular, near the tip of the Antarctic Peninsula, have yielded a great variety of plant and animal fossils from Late Cretaceous and early Cenozoic times (100-34 Ma ago) (Francis et al., 2008a[3]). In addition, deep drilling into marine sediments on the continental margin of Antarctica (Figure 3.1) has provided records of Antarctica’s climate during the tens of millions of years that it has been ice-covered (Barrett, 2009).
Deep drilling for direct records of Antarctic climate history has taken place in only a few locations around the margin (e.g. ship-based drilling in the Antarctic Peninsula, Prydz Bay and Ross Sea, and drilling from sea- and shelf-ice platforms in McMurdo Sound). In contrast, the collection of relatively short piston cores and vibracores of sediments from the seas around Antarctica has been widespread. These cores, typically less than 10 m long, but on occasion up to 40 m, provide typically late Quaternary sequences that record changes in, for example, the duration or extent of sea ice, the proximity and stability of glaciers and ice shelves, changing oceanic temperature, surface water productivity, and clues to the behaviour of ocean currents (Anderson, 1999[4]). In addition, collections of deep-sea drill cores from the wider world ocean provide information from oxygen isotopes in plankton shells about the water temperature, and information from carbon isotopes about the water flux. This can be linked to production of cold deep bottom water from Antarctica and changes in the global signature of ice volume, which we can use to interpret the role of Antarctica in the global climate system over time (Kennett, 1982[5]; Miller et al., 2008[6]; Zachos et al., 2008[7]).
Ice cores provide a continuous high-resolution record of environmental change on timescales of up to c. 800 ka. Here water molecules, soluble and insoluble chemistry, particles, gases and clathrates trapped in the ice provide valuable archives of change in past atmospheric composition, temperature, precipitation, major trace chemistry and other environmental parameters such as changes in atmospheric circulation, sea ice extent, and volcanic activity. They offer the possibility of improving knowledge of key climate forcings (solar, volcanic) and of key climate feedbacks (greenhouse gases, aerosols). The ice core data are also crucial in benchmarking climate and ice sheet models against observations. The longest ice core records date back over 800 ka (Jouzel et al., 2007[8]). However, from marine sediments, we know that just before this time the pattern of climate variability was different, with glacial cycles of only 40 ka length, compared with the 100 ka cycles today. If we are to understand the natural variability that has led us to our current climate, it is critical to understand what caused the length of the cycles to change. It is therefore a key challenge to identify the locations where ice older than 1 Ma can be found (IPICS, 2008[9]) in order to test the hypothesis that the change from 40 ka to 100 ka cycles was caused by a lowering of atmospheric carbon dioxide concentrations. This is because the longer ice cores enable assessment of the natural relationship between greenhouse gases and climate, allowing us to deduce the underlying rules governing climate including the processes that control exchange of carbon dioxide between reservoirs. Many ice core studies also focus on the last 40 ka which includes the transition from the last glacial into the present warm interglacial and a sequence of abrupt swings in climate which are particularly apparent in Greenland ice cores and other climate archives, and to a lesser extent in Antarctic ice cores. This glacial-interglacial transition is the best documented global response to very large-scale changes in climate boundary conditions, and the ice cores enable researchers to reconstruct the precise sequence of events (including forcings and responses) through the last glacial-interglacial transition and related changes in greenhouse gas concentrations, accumulation and ice sheet extent, ocean surface conditions (e.g. sea ice and marine biological productivity) and changing thermohaline circulation patterns (IPICS, 2008[9]).
Glacial geomorphological records such as moraines and trimlines, dated using cosmogenic isotopes from exposed rocks, also provide information on past ice sheet extent and thickness, and the timing of glaciation and deglaciation extending back from tens of thousands to millions of years (e.g. Summerfield et al., 1999[10]; Mackintosh et al., 2007[11]; Jamieson and Sugden, 2008[12]). On land, the drilling of sediments that have accumulated in lakes in the ice-free regions of Antarctica provides geological records of climate that typically span just the present Holocene interglacial (last 11.7 ka), the most recent period of relatively stable global climate, and a time that has seen the rise of human civilizations. These sediments contain detailed and high resolution records of changes in temperature-related variables such as lake ice cover, moisture balance, biological productivity, ecology and species composition, together with records of deglaciation, isostasy and relative sea level change (Hodgson et al., 2004a[13]).
Using geological records, the next section describes the geological setting of Antarctica and its features during “greenhouse” times, the changes as dynamic ice sheets grew and pulsated from orbital forcing, and the shift to a colder more persistent East Antarctic Ice Sheet 14 million years ago and finally the stabilisation of the West Antarctic Ice Sheet in the last million years.
Pages in this topic
- Deep time
- The last million years
- The Holocene
- Biological responses to climate change
- Concluding remarks on the pre-instrumental period
References
- ↑ Naish, T.R., Carter, L., Wolff, E. and Powel, R.D. 2008a. Chapter 11 - Late Pliocene-Pleistocene Antarctic climate variability at orbital and suborbital scale: Ice sheet, ocean and atmospheric interactions. In: Florindo F, Siegert M (eds) Antarctic Climate Evolution, Developments in Earth and Environmental Science, 8, Elsevier, Amsterdam.
- ↑ Barrett, P.J. 1996. Antarctic palaeoenvironment through Cenzoic times - a review, Terra Antartica, 3, 103-119.
- ↑ Francis, J.E., Ashworth, A., Cantrill, D.J., Crame, J.A., Howe, J., Stephens, R., Tosolini, A-M. and Thorn, V. 2008a. 100 million years of Antarctic climate evolution: evidence from fossil plants. In: Cooper AK, Barrett PJ, Stagg H, Storey B, Stump E, Wise W (eds) Antarctica: A Keystone in a Changing World. Proceedings of the 10th International Symposium on Antarctic Earth Sciences. The National Academies Press, Washington, DC, 19-27.
- ↑ Anderson, J.B. 1999. Antarctic marine geology, Cambridge University Press, Cambridge, UK.
- ↑ Kennett, J.P. 1982. Marine Geology. Prentice Hall,, Englewood Cliffs, 813 pp.
- ↑ Miller, K.G., Wright, J.D., Katz, M.E., Browning, J.V., Cramer, B.S., Wade, B.S. and Mizintseva, S.F. 2008. A View of Antarctic Ice-Sheet Evolution from Sea-Level and Deep-Sea Isotope Changes During the Late Cretaceous-Cenozoic. In: Cooper AK, Barrett P, Stagg H, Storey B, Stump E, Wise W (eds) Antarctica: A Keystone in a Changing World. Proceedings of the 10th International Symposium on Antarctic Earth Sciences, Santa Barbara, California, August 26 to September 1, 2007. Polar Research Board, National Research Council, U.S. Geological Survey.
- ↑ Zachos, J.C., Dickens, G.R. and Zeebe, R.E. 2008. An early Cenozoic perspective on greenhouse warming and carbon-cycle dynamics, Nature, 451, 279-283.
- ↑ Jouzel, J., Masson-Delmotte, V., Cattani, O., Dreyfus, G., Falourd, S., Hoffmann, G., Minster, B., Nouet, J., Barnola, J.M., Chappellaz, J., Fischer, H., Gallet, J.C., Johnsen, S., Leuenberger, M., Loulergue, L., Luethi, D., Oerter, H., Parrenin, F., Raisbeck, G., Raynaud, D., Schilt, A., Schwander, J., Selmo, E., Souchez, R., Spahni, R., Stauffer, B., Steffensen, J.P., Stenni, B.S., Tison, J.L., Werner, M. and Wolff, E. 2007. Orbital and millenial Antarctic climate variability over the past 800,000 years, Science, 317, 793-796.
- ↑ 9.0 9.1 IPICS 2008. International Partnerships in Ice Core Sciences - white papers. In: Wolff E, Brook E (eds). http://www.pages.unibe.ch/ipics/steeringcommittee.html.
- ↑ Summerfield, M.A., Stuart, F.M., Cockburn, H.A.P., Sugden, D.E., Denton, G.H., Dunai, T. and Marchart, D. R. 1999. Long-term rates of denudation in the Dry Valleys, Transantarctic Mountains, southern Victoria Land, Antarctica, based on in-situ-produced cosmogenic 21Ne, Geomorphology, 27, 113-129.
- ↑ Mackintosh, A., White, D., Fink, D., Gore, D.B., Pickard, J. and Fanning, P.C. 2007. Exposure ages from mountain dipsticks in Mac. Robertson Land, East Antarctica, indicate little change in ice-sheet thickness since the Last Glacial Maximum, Geology, 35, 551-554.
- ↑ Jamieson, S.R. and Sugden, D.E. 2008. Landscape Evolution of Antarctica. in: Cooper AK, Barrett P, Stagg H, Storey B, Stump E, Wise W (eds) Antarctica: A Keystone in a Changing World. Proceedings of the 10th International Symposium on Antarctic Earth Sciences, Santa Barbara, California, August 26 to September 1, 2007. Polar Research Board, National Research Council, U.S. Geological Survey
- ↑ Hodgson, D.A., Doran, P.T., Roberts, D. and McMinn, A. 2004a. Paleolimnological studies from the Antarctic and subantarctic islands. In: Pienitz R, Douglas MSV, Smol JP (eds) Developments in Palaoenvironmental Research. Long-term Environmental Change in Arctic and Antarctic Lakes, 8, Springer, Dordrecht, 419-474.