The Southern Ocean in the instrumental period
- This page is part of the topic Antarctic climate and environment change in the instrumental period
Introduction
At first sight the Southern Ocean seems to be dominated by circumpolar symmetry mainly assured by the circumpolar current bands of the eastward flowing ACC covering mainly the mid latitudes (Figure 1.8) and the westward flowing Antarctic Coastal Current surrounding the continental margin in high latitudes. Superimposed on the zonal flow there are meridional circulation cells (Figure 1.9), the deep one related to sinking motion along the continental slope to form the Antarctic Bottom Water, and the shallow one forming Mode and Intermediate Waters in the mid latitudes. In spite of the current system linking the Atlantic, Indian and Pacific sectors, significant zonal differences are obvious and require a regional description of the variability and change in the Southern Ocean. Differences are related to the shape of the ocean basins and ridges which strongly affect the ocean currents, the shape of the continent with huge embayments, such as the Weddell and the Ross Seas giving rise to subpolar gyres (Figure 1.10), the distributions of ice shelves, the zonal structure of the forcing (storm tracks) and finally the different hydrographic conditions in the northward adjacent ocean basins.
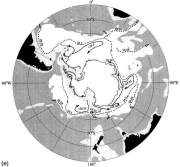
The meridional circulation cells which can only schematically be represented in a two dimensional way (Figure 1.9), display clear zonal differences as well. They are obvious in the long term mean conditions, e.g. the horizontal distribution of the newly formed bottom water (Figure 4.22, Orsi et al., 1999[1]) which result in the variable contributions of different sectors to the bottom water formation. It is estimated that 66% can be contributed to originate in the Weddell Sea, 25% in the Australian Sector and 7% in the Ross Sea (Rintoul, 1998[2]). Due to the zonal differences in the hydrographic and forcing conditions, variations differ in the sectors as well, e.g. cooling and freshening of the newly formed bottom water in the Australian sector (Rintoul, 2007[3]) and warming and increase of salinity of bottom water on the prime meridian (Fahrbach et al., 2004[4]). However, even within the basins different patterns are detected e.g. in the Weddell Sea (Fahrbach et al., 2004[4]). To take into account the circumpolar differences in the observed variations the description of the Southern Ocean is arranged here zonally in different sectors. Since regional differences occur even on smaller scales than the three large ocean sectors, we focus on sub-areas where a particular type of variation is observed.
There is evidence that changes in the Antarctic Bottom Water propagate into the global ocean. Warming of the northward flow of Antarctic Bottom Water on a decadal time scale is observed in Vema Channel from the Argentine to the Brazil Basin (Zenk and Morozov, 2007[5]). Large scale abyssal warming over decades is observed in the South Atlantic (Johnson and Doney, 2006[6]) and the Pacific (Johnson et al., 2007[7]).
Observation of change in surface waters is difficult to detect since there is an intensive seasonal cycle and only few observations. However, around South Georgia observations exist since 1925, which are frequent enough to resolve the annual cycle. They reveal significant trends in the upper 150 m, with 2.3°C of warming over 81 years, which are twice as strong in winter than in summer (Whitehouse et al., 2008[8]).
Sub-Antarctic Mode Water is formed by winter cooling and convection just north of the Sub-Antarctic Front (McCartney, 1977[9], 1982[10]). When it is subducted it becomes Antarctic Intermediate Water (Hanawa and Talley, 2001[11]). This water mass is of particular interest due to its capacity to take up atmospheric CO2 (Sabine et al., 2004[12]). Significant warming (Wong, 2001; Gille, 2002[13]; Aoki et al., 2003[14]) and freshening was observed in these water masses (Wong et al., 1999[15]; Curry et al., 2003[16]; Bindoff et al., 2007[17]; Böning et al., 2008[18]) over the last decades. To understand the changes, the formation processes (e.g. Sallée et al., 2006[19]) and the turbulent character of the field (Tomczak, 2007[20]) need to be understood.
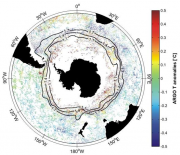
In a circumpolar view the ACC band has warmed in recent decades (Figure 4.23; Gille, 2002[13], 2008[21]; Levitus et al., 2000[22], 2005[23]; Böning et al., 2008[18]). The changes are consistent with a southward shift of the ACC (Gille, 2008[21]). Some climate models suggest that the ACC shifts south in response to a southward shift of the westerly winds driven by enhanced greenhouse forcing (Fyfe and Saenko, 2006[24]; Bi et al., 2002[25]). A significant part of the changes in the wind system can be related to the positive trend in the SAM (e.g. Hughes et al., 2003[26]). The poleward shift and intensification of winds over the Southern Ocean has been attributed to both changes in ozone in the Antarctic stratosphere (Thompson and Solomon, 2002[27]) and to greenhouse warming (Fyfe et al., 1999[28]). In addition to driving changes in the ACC, the wind changes have caused a southward expansion of the subtropical gyres (Cai, 2006[29]) and an intensification of the Southern Hemisphere “supergyre” that links the three subtropical gyres (Speich et al., 2002[30], 2007[31]). The “supergyre” provides the mechanism by which Sub-Antarctic Mode Water and Antarctic Intermediate Water is distributed between the ocean basins (Ridgway and Dunn, 2007[32]). In the area of the formation of Antarctic Intermediate Water north of the Subantarctic Front freshening is observed. Surprisingly the changes are rather similar all along the ACC (Figure 4.23; Böning et al., 2008[18]).
Pages in this topic
- The Australian sector in the instrumental period
- The Amundsen and Bellingshausen seas in the instrumental period
- Ross Sea shelf waters in the instrumental period
- The Weddell Sea sector in the instrumental period
- Small-scale processes in the Southern Ocean
- Modelling of the ACC and polar gyres
References
- ↑ 1.0 1.1 Orsi, A.H., Johnson, G.C. and Bullister, J.B. 1999. Circulation, mixing and production of Antarctic Bottom Water, Prog. Oceanog., 43, 55-109.
- ↑ Rintoul, S.R. 1998. On the origin and influence of Adelie Land Bottom Water. In: Ocean, Ice and Atmosphere: Interactions at the Antarctic Continental Margin, S. Jacobs and R. Weiss (eds.), Antarctic Research Series, 75, 151-171, American Geophysical Union, Washington.
- ↑ Rintoul, S.R. 2007. Rapid freshening of Antarctic Bottom Water formed in the Indian and Pacific Oceans, Geophys. Res. Lett., 34, L06606, doi:10.1029/2006GL028550.
- ↑ 4.0 4.1 Fahrbach, E., Hoppema, M., Rohardt, G., Schröder, M. and Wisotzki, A. 2004. Decadal-scale variations of water mass properties in the deep Weddell Sea, Ocean Dynamics, 54, 77-91.
- ↑ Zenk, W. and Morozov, E. 2007. Decadal warming of the coldest Antarctic Bottom Water flowing through the Vema Channel, Geophysical Research Letters, 34, L14607, doi:10.1029/2007/GLR030340
- ↑ Johnson, G.C. and Doney, S.C. 2006. Recent western South Atlantic bottom water warming, Geophysical research Letters, 33, L14614, doi:10.1029/2006GL026769.
- ↑ Johnson, G.C., Mecking, S., Sloyan, B.M. and Wijffels, S.E. 2007. Recent Bottom Water Warming in the Pacific Ocean, Journal of Climate, 5365-5375, doi:10.1175/2007JCLI1879.1.
- ↑ Whitehouse, M.J., Meredith, M.P., Rothery, P., Atkinson, A., Ward, P. and Korb, R.E. 2008. Rapid warming of the ocean at South Georgia, Southern Ocean during the 20th Century: forcings, characteristics and implications for lower trophic levels, Deep-Sea Research I, 55, 1218-1228.
- ↑ McCartney, M.S. 1977. Subantarctic Mode Waters. In: Angel, M. (ed) A voyage of discovery, Pergamon, New York, 103-119.
- ↑ McCartney, M.S. 1982. The subtropical circulation of Mode Waters, Journal of Marine Research, 40 (suppl), 427-464.
- ↑ Hanawa, K. and Talley, L.D. 2001. Mode Waters. In: Eds. G. Siedler, J. Church and J. Gould, Ocean circulation and climate; observing and modelling the global ocean, International Geophysics Series, 77, 373-386, Academic Press.
- ↑ Sabine, C.L., ET AL. 2004. The Oceanic Sink for Anthropogenic CO2, Science, 305, 367-371.
- ↑ 13.0 13.1 Gille, S.T. 2002. Warming of the Southern Ocean since the 1950s, Science, 295(5558), 1275-1277, doi:10.1126/science.1065863.
- ↑ Aoki, S., Nakazawa, T., Machida, T., Sugawara, S., Morimoto, S., Hashida, G., Yamanouchi, T., Kawamura, K. and Honda, H. 2003. Carbon Dioxide Variations in the Stratosphere Over Japan, Scandinavia and Antarctic, Tellus, 55B, 178-186.
- ↑ Wong, A.P.S., Bindoff, N.L. and Church, J.A. 1999. Large-scale freshening of intermediate waters in the Pacific and Indian oceans, Nature, 400, 86743), 440-443.
- ↑ Curry, R., Dickson, B. and Yashayaev, I. 2003. A change in the freshwater balance of the Atlantic Ocean over the past decades, Nature, 426(6968), 826-829.
- ↑ Bindoff, N., Willebrand, J., Artale, V., Cazenave, A., Gregory, J., Gulev, S., Hanawa, K., Le Quéré, C., Levitus, S., Nojiri, Y., Shum, C., Talley, L. and Unnikrishnan, A. 2007. Observations: oceanic climate change and sea level. In Climate Change 2007: The Physical Science Basis. Contribution of Working Group 1 to the Fourth Assessment Report of the Intergovernmental Panel on Climate Change (eds. S. Solomon, D. Qin, and M. Manning). Cambridge University Press. United Kingdom and New York, NY, USA.
- ↑ 18.0 18.1 18.2 18.3 Böning, C. W., A. Dispert, M. Visbeck, S. R. Rintoul, and F. Schwarzkopf, 2008. Observed multi-decadal ocean warming and density trends across the Antarctic Circumpolar Current, Submitted.
- ↑ Sallée, J.-B., Wienders, N., Speer, K. and Morrow, R. 2006. Formation of subantarctic mode water in the southeastern Indian Ocean, Ocean Dynamics, 56, 525-542, DOI 10.1007/S10236-005-0054-X.
- ↑ Tomczak, M. 2007. Variability of Antarctic intermediate Water properties in the South Pacific Ocean, Ocean Science, 3, 363-377.
- ↑ 21.0 21.1 Gille, S.T. 2008. Decadal-scale temperature trends in the Southern Hemisphere ocean, J. Clim., 21(18), 4749-4765.
- ↑ Levitus, S., Antanov, J.I., Boyer, T.P. and Stephens, C. 2000. Warming of the world ocean, Science, 287 (5461), 2225-2229.
- ↑ Levitus, S., Antonov, J. and Boyer, T. 2005. Warming of the world ocean, Geophysical Res. Letters, 32, L02604, doi:10.1029/2004GL021592.
- ↑ Fyfe, J.C. and Saenko, O.A. 2006. Simulated changes in extratropical Southern Hemisphere winds and currents, Geophys. Res. Letters, 33, L06701, doi:10.1029/2005GL025332.
- ↑ Bi, D., Budd, W.F., Hirst, A.C. and Wu, X. 2002. Response of the Antarctic circumpolar current transport to global warming in a coupled model, Geophys. Res. Lett., 29 (24), 2173, doi:10.1029/2002GL015919.
- ↑ Hughes, C.W., Woodworth, P.L., Meredith, M.P., Stepanov, V., Whitworth, T. and Pyne A.R. 2003. Coherence of Antarctic sea levels, Southern Hemisphere Annular Mode, and flow through Drake Passage, Geophysical Research Letters, 30(9), 1464, doi:10.1029/2003GL017240.
- ↑ Thompson, D. and Solomon, S. 2002. Interpretation of recent southern hemisphere climate change, Science, 296(5569), 895-899.
- ↑ Fyfe, J.C., Boer, G.J. and Flato, G.M. 1999. The Arctic and Antarctic oscillations and their projected changes under global warming, Geophys. Res. Lett., 26, 1601-1604.
- ↑ Cai, W. 2006. Antarctic ozone depletion causes in intensification of the Southern Ocean super-gyre circulation, Geophys. Res. Lett., 33, doi:10.1029/2005GL024911.
- ↑ Speich, S., Blanke, B., De Vries, P., ET AL. 2002. Tasman leakage: A new route in the global ocean conveyor belt, Geophys. Res. Lett., 29, 1416.
- ↑ Speich, S., Blanke, B. and Cai, W.J. 2007. Atlantic meridional overturning circulation and the Southern Hemisphere supergyre, Geophys. Res. Let., 34, L23614.
- ↑ Ridgway, K.R. and Dunn, J.R. 2007. Observational evidence for a Southern Hemisphere oceanic supergyre, Geophysical Research Letters, 34, L13612, doi:10.1029/2007GL030392.