The Greenhouse world - from the breakup of Gondwana to 34 million years ago
- This page is part of the topic Deep time
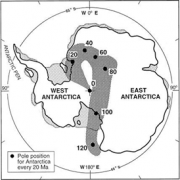
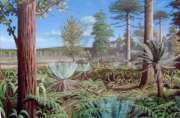
Some 200 million years ago (Ma) Antarctica was the centrepiece of the Gondwana super-continent, which began to break up around 180 Ma in the Jurassic Period of the Mesozoic Era. The Gondwanan fragments separated by sea-floor spreading largely between around 100 to 65 Ma, during the Cretaceous Period, although by this time Antarctica had already moved into a position over the South Pole (Figure 3.2). A rich record of plant and animal fossils from Late Cretaceous and early Tertiary times has been found on Seymour Island and Alexander Island in the Antarctic Peninsula. This demonstrates that, despite its polar position, Antarctica had a warm temperate climate that allowed the growth of lush forests (Figure 3.3). These were inhabited by dinosaurs in the Cretaceous and mammals during the early Tertiary (Francis and Poole, 2002[3]).
Analysis of changing biodiversity in the forests, and of the climate record preserved in the foliage, indicates that climate cooling signalled the decline of Antarctic warmth during the Middle Eocene, about 45 Ma, when heat-loving plants were lost from Antarctica and replaced by types such as species of Nothofagus trees that could tolerate cold climates (Francis et al., 2008a[2]).
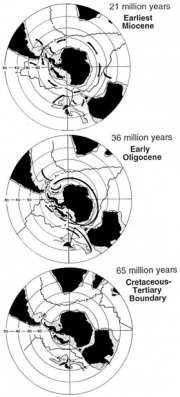
Over time, South America, Africa, India, Australia and New Zealand moved away from Antarctica, opening the South Atlantic, Indian and Southern Oceans (Figure 3.4). As the proto-Pacific Plate was subducted beneath the Antarctic Plate an active volcanic arc grew, the eroded remnants of which now form the Antarctica Peninsula. The western margin of Antarctica was thus characterised by volcanism and lateral movement of fragments of the formerly continuous Gondwana terrain (for example see McCarron and Larter, 1998[5]). This western arc broke apart first at around 85 Ma south of New Zealand, but much later between South America and the Antarctic Peninsula to create the Drake Passage.
Over the last 40 million years, the Antarctic shelf experienced a series of tectonic, climatic and oceanographic events that lead to isolation from other oceans, establishment of colder conditions and complete replacement and impoverishment of the fish fauna. The deepening of the separations from other continental masses and the removal of the last barriers to circumpolar flow, allowed the establishment of the Antarctic Circumpolar Current (ACC) and, at its northern border, of the Antarctic Polar Front (APF), a roughly circular oceanic system running between 50°S and 60°S and extending to 2,000 m in depth.
Two key events allowed the establishment of the ACC: (1) the opening of the Tasman Seaway between Antarctica and Australia, which according to tectonics and marine geology occurred approximately 35.5-30 Ma (Kennett, 1977[6]; Lawver and Gahagan, 2003[7]; Stickley et al., 2004[8]; Wei, 2004[9]); (2) the opening of the Drake Passage between southern South America and the Antarctic Peninsula. The two openings allowed the development of the ACC. The timing of the opening of the Passage is controversial, with estimates ranging between 40 and 17 Ma (Barker and Burrell, 1977[10]; Livermore et al., 2004[11], Scher and Martin, 2006[12]). Such a relatively wide time window is due to contrasting views on the palaeo-elevation of key parts of the Scotia Arc and the Shackleton Fracture Zone (reviewed in Barker et al., 2007[13]). Recently, using neodymium isotopes to detect the presence of Pacific seawater in the Atlantic sector Scher and Martin (2006[12]) proposed that the opening of the Drake passage occurred as early as 41 Ma, and was thus completed before the establishment of the Tasman Seaway.
The timing of the final onset of the ACC is also uncertain (Barker et al., 2007[13]). Estimates from sediment records range from late Eocene (40 Ma) to latest Miocene (8-10 Ma) (Wei and Wise, 1992[14]; Scher and Martin, 2006[12]). Concerns have been raised that some of these estimates could rather be climate-related and reflect a change in either temperature or productivity independent of ocean circulation (Barker et al., 2007[13]). The older view that the ACC developed around the Eocene-Oligocene boundary leading to the first continental ice sheets on Antarctica by reducing heat transport into the region (Kennett, 1977[6]) has been questioned by DeConto and Pollard (2003[15]). Their modelling study shows the first ice sheets could have formed from a drop in atmospheric CO2 levels from 3 to 2 times pre-industrial levels and that the opening of Drake Passage could provide only 20% of the cooling. The formation of the circum-polar deep water current may have subsequently helped the intensification of glaciation (Francis et al., 2008b[16]).
Despite the uncertainty on both the timing of final onset of the ACC and its importance as a driver of climatic change, palaeoclimatic reconstructions leave little doubt about the fact that oceanographic and climatic processes responsible for current day glacial conditions in the Antarctic started in the same period of time. In fact, switching of climate conditions from “greenhouse” to “icehouse” in the Antarctic started in the late Eocene, 42 Ma, with an initial phase of strong but short-lived glaciations that match with the most recently published time estimates of formation of the Drake passage (Scher and Martin, 2006[12]). A further drastic change occurred at the Eocene-Oligocene boundary ~34 Ma, (Tripati et al., 2005[17]; Barker et al., 2007[13]), leading to ice-sheet coverage similar in extent to the present ice sheet and contemporaneous with the opening of the Tasman Seaway.
The palaeontological record suggests these climatic events also influenced the Southern Ocean and its fish fauna. The best studied Antarctic fish fossils have been discovered in La Meseta Formation on Seymour Island (at the tip of the Antarctic Peninsula). The deposit from the late Eocene showed a fauna still “cool and temperate in character” that possibly lived in waters such as those found today around Tasmania, New Zealand and southern South America (Eastman, 2005[18]). Accordingly, the earliest cold-climate marine Antarctic faunas are thought to date back to the latest Eocene-Oligocene (35 Ma).
With the ACC, the Southern Ocean became the cold isolated habitat that we know today. However, ice sheet coverage oscillated throughout the Cenozoic and probably produced repeated shifts of species distribution in Antarctic coastal waters, allowing allopatric speciation and diversification of Antarctic taxa (Rogers, 2007[19]). In addition, the present APF is now suspected to be “leaky” and to allow transport of plankton north in mesoscale eddies with cold cores (Clarke et al., 2005[20]).
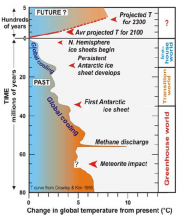
Atmospheric CO2 levels ranged from ~3,000 ppm in the Early Cretaceous (at 130 Ma) to around 1,000 ppm in the Late Cretaceous (at 70 Ma) and Early Cenozoic (at 45 Ma) (e.g. Pagani et al., 2005[25]; Royer, 2006[26]), leading to global temperatures at least 6 or 7ºC warmer than present at times. These high CO2 levels were probably the consequence of volcanic outgassing. Temperatures peaked at ~85 Ma during the mid-Late Cretaceous when sub-tropical climates prevailed over the pole (Francis et al., 2008a[2]). Even in this Cretaceous greenhouse world from oxygen isotope and backstripping data some researchers have inferred sea level changes in the order of tens of metres and intermittent polar ice sheets (Miller et al., 2008[27]), although there is as yet no geological evidence (Thorn et al., 2007[28]). Temperatures peaked again at 50 Ma (Figure 3.5) and subsequently declined, as did atmospheric CO2 levels (Pearson and Palmer, 2000[29]; Pagani et al., 2005[25]; Royer, 2006[26]). Superimposed on the high CO2 world of the Early Cenozoic, deep-sea sediments provide evidence of the catastrophic release of more than 2,000 gigatonnes of carbon into the atmosphere from methane hydrates around 55 Ma ago, at the Paleocene-Eocene boundary, raising global temperatures by a further ~4-5°C, although they recovered after about 100,000 years (Figure 3.5) (Zachos et al., 2003[22]; Zachos et al., 2005[23]).
References
- ↑ Divenere, V.J., Kent, D.V. and Dalziel, I.W.D. 1994. Mid-Cretaceous paleomagnetic results from Marie Byrd Land, WestAntarctica: a test of post-100 Ma relative motion between East and West Antarctica, Journal of Geophysical Research, 99, 15115-15139.
- ↑ 2.0 2.1 2.2 Francis, J.E., Ashworth, A., Cantrill, D.J., Crame, J.A., Howe, J., Stephens, R., Tosolini, A-M. and Thorn, V. 2008a. 100 million years of Antarctic climate evolution: evidence from fossil plants. In: Cooper AK, Barrett PJ, Stagg H, Storey B, Stump E, Wise W (eds) Antarctica: A Keystone in a Changing World. Proceedings of the 10th International Symposium on Antarctic Earth Sciences. The National Academies Press, Washington, DC, 19-27.
- ↑ Francis, J.E. and Poole, I. 2002. Cretaceous and early Tertiary climates of Antarctica : evidence from fossil wood, Palaeogeography, Palaeoclimatology, Palaeoecology, 182, 47-64.
- ↑ Kennett, J.P. 1978. The development of planktonic biostratigraphy in the Southern Ocean during the Cenozoic, Marine Micropaleontology, 3, 301-345.
- ↑ McCarron, J.J. and Larter, R.D. 1998. Late Cretaceous to Early Tertiary Subduction History of the Antarctic Peninsula, J. Geol. Soc. Lond., 155, 255-268.
- ↑ 6.0 6.1 Kennett, J.P. 1977. Cenozoic evolution of the Antarctic glaciation, the circum-Antarctic ocean and their impact on global paleoceanography, J. Geophys. Res., 82, 3843-3876.
- ↑ Lawver, L.A. and Gahagan, L.M. 2003. Evolution of Cenozoic seaways in the circum-Antarctic region, Palaeogeography, Palaeoclimatology, Palaeoecology, 198, 11-37.
- ↑ Stickley, C.E., Brinkhuis, H., Schellenberg, S.A., Sluijs, A., Röhl, U., Fuller, M., Grauert, M., Huber, M., Warnaar, J. and Williams, G.L. 2004. Timing and nature of the deepening of the Tasmanian Gateway, Paleoceanography, 19, PA4027, doi:4010.1029/2004PA001022.
- ↑ Wei, W. 2004. Opening of the Australia-Antarctica Gateway as dated by nannofossils, Marine Micropaleontology, 52, 133-152.
- ↑ Barker, P.F. and Burrell, J. 1977. The opening of Drake passage, Marine Geology, 25.
- ↑ Livermore, R., Nankivell, A., Eagles, G. and Morris, P. 2004. Paleogene opening of Drake Passage, Earth and Planetary Science Letters, 236, 459-470.
- ↑ 12.0 12.1 12.2 12.3 Scher, H.D. and Martin, E.E. 2006. Timing and Climatic Consequences of the Opening of Drake Passage, Science, 312, 428-430.
- ↑ 13.0 13.1 13.2 13.3 Barker, P.F., Diekmann, B. and Escutia, C. 2007. Onset of Cenozoic Antarctic Glaciation, Deep Sea. Research, 54, 2293-2307.
- ↑ Wei, W. and Wise, S.W. 1992. Eocene-Oligocene calcareous nannofossil magnetobiochronology of the Southern Ocean, Newsl. Stratigr., 26, 119-132.
- ↑ Deconto, R.M. and Pollard, D. 2003. Rapid Cenozoic glaciation of Antarctica induced by declining atmospheric CO2, Nature, 421, 245-249.
- ↑ Francis, J.E., Marenssi, S., Levy, R., Hambrey, M., Thorn, V.T., Mohr, B., Brinkhuis, H., Warnaar, J., Zachos, J., Bohaty, S. and Deconto, R. 2008b. From Greenhouse to Icehouse - The Eocene/Oligocene in Antarctica. In: Florindo F, Siegert M (eds) Antarctic Climate Evolution, Developments in Earth and Environmental Science, Vol 8. Elsevier, Amsterdam.
- ↑ Tripati, A., Backman, J., Elderfield, H. and Ferretti, P. 2005. Eocene bipolar glaciation associated with global carbon cycle changes, Nature, 436, 341-346.
- ↑ Eastman, J.T. 2005. The nature of the diversity of Antarctic fishes, Polar Biology, 28, 1432-2056.
- ↑ Rogers, A.D. 2007. Evolution and biodiversity of Antarctic organisms: a molecular perspective, Philosophical Transactions of the Royal Society of London, Series B, Biological Sciences, 362, 2191-2214.
- ↑ Clarke, A., Barnes, D.K.A. and Hodgson, D.A. 2005. How isolated is Antarctica? Trends in Ecology and Evolution, 20, 1-3.
- ↑ Crowley, T.J. and Kim, K. 1995. Comparison of long-term greenhouse projections with the Geologic Record, Geophysical Research Letters, 22, 933-936.
- ↑ 22.0 22.1 Zachos, J.C., Wara, M.W., Bohaty, S., Delaney, M.L., Petrizzo, M.R., Brill, A., Bralower, T.J. and Premoli-Silva, I. 2003. A Transient Rise in Tropical Sea Surface Temperature During the Paleocene-Eocene Thermal Maximum, Science, 302, 1551-1554.
- ↑ 23.0 23.1 Zachos, J.C., Rohl, U., Schellenberg, S.A., Sluijs, A., Hodell, D.A., Kelly, D.C., Thomas, E., Nicolo, M., Raffi, I., Lourens, L.J., McCarren, H. and Kroon, D. 2005. Rapid Acidification of the Ocean During the Paleocene-Eocene Thermal Maximum, Science, 308, 1611-1615.
- ↑ Mayewski, P.A., Meredith, M.P., Summerhayes, C.P., Turner, J., Worby, A.P., Barrett, P.J., Casassa, G., Bertler, N.A.N., Bracegirdle, T.J., Naveira-Garabato, A.C., Bromwich, D.H., Campbell, H., Hamilton, G.H., Lyons, W.B., Maasch, K.A., Aoki, S., and Xiao, C. 2009. State of the Antarctic and Southern Ocean Climate System (SASOCS), Reviews of Geophysics, 47, RG1003, doi:10.1029/2007RG000231.
- ↑ 25.0 25.1 Pagani, M., Zachos, J.C., Freeman, K.H., Tipple, B. and Bohaty, S. 2005. Marked Decline in Atmospheric Carbon Dioxide Concentrations During the Paleogene, Science, 309, 600-603.
- ↑ 26.0 26.1 Royer, D.L. 2006. CO2-forced climate thresholds during the Phanerozoic, Geochim. Cosmochim. Acta, 70, 5665-5675.
- ↑ Miller, K.G., Wright, J.D., Katz, M.E., Browning, J.V., Cramer, B.S., Wade, B.S. and Mizintseva, S.F. 2008. A View of Antarctic Ice-Sheet Evolution from Sea-Level and Deep-Sea Isotope Changes During the Late Cretaceous-Cenozoic. In: Cooper AK, Barrett P, Stagg H, Storey B, Stump E, Wise W (eds) Antarctica: A Keystone in a Changing World. Proceedings of the 10th International Symposium on Antarctic Earth Sciences, Santa Barbara, California, August 26 to September 1, 2007. Polar Research Board, National Research Council, U.S. Geological Survey.
- ↑ Thorn, V.C., Francis, J.E., Riding, J.B., Raiswell, R.W., Pirrie, D., Haywood, A.M., Crame, J.A. and Marshall, J.M. 2007. Terminal Cretaceous climate change and biotic response in Antarctica In: Online Proceedings of the 10th ISAES X, USGS Open-File Report 2007-1047, Extended Abstract 096, 4 p.
- ↑ Pearson, P.N. and Palmer, M.R. 2000. Atmospheric carbon dioxide concentrations over the past 60 million years, Nature, 406, 695-699.