Terrestrial biological responses to climate change
- This page is part of the topic Biological responses to climate change
With the exception of lake sediment studies (Hodgson et al., 2004a[1]), little terrestrial research has set out to examine changes in biodiversity, distributions and abundance over Holocene and longer timescales. There has been a widely held but untested general assumption that, owing to the much more extensive and thicker ice sheets present on the continent at LGM, many if not all contemporary Antarctic terrestrial biota must be recent colonists. That this is not the case has come to recent prominence (Convey and Stevens, 2007[2]; Convey et al., 2008[3]; Pugh and Convey, 2008[4]), and it is becoming clear from biogeographical and molecular evidence that across the continent and also the sub-Antarctic islands the contemporary biota is a result of vicariance and colonization processes that have taken place on all timescales between pre-LGM and pre-Gondwana-breakup (e.g. Stevens et al., 2006[5]). Nevertheless it is also clear that much of the tiny proportion of Antarctica that is ice-free today has been exposed over only the last few thousand years during post LGM glacial retreat. Through the maritime Antarctic and much of the continental coastline, most exposed ground takes the form of ‘islands’ of terrestrial habitat of varying size at low altitude and close to the coast, surrounded by either hostile sea or ice (Bergstrom and Chown, 1999[6]). Exceptions to this generalization are provided first by the continental Antarctic ‘Dry Valleys’ of Victoria Land, providing several thousand square kilometres of ground at least some of which has been continuously exposed since about 12 Ma in the Miocene, and second by inland higher altitude nunataks and mountain ranges, some of which will not have been covered at Pleistocene glacial maxima (Stevens and Hogg, 2003[7]; Stevens et al., 2006[5]).
Taking the maritime Antarctic as an example, it is thus clear (a) that the large majority of areas of currently ice free ground have been exposed post LGM (while longer term refugia are required to explain contemporary biota distributions, their precise locations remain unknown (Hodgson and Convey, 2005[8]; Pugh and Convey, 2008[4])), and at the same time (b) it is clear that most elements of the regional biota have successfully colonized those areas that have been exposed, and done so rapidly, as their terrestrial communities are in most cases entirely typical of this regional biota. Hodgson and Convey (2005[8]), using terrestrial arthropod abundances obtained from lake sediment cores on maritime Antarctic Signy Island, identified some differences in relative abundances of two common mite species over time (the last 5+ ka) that they proposed to be consistent with climatic changes that would have altered the balance of the different habitats that these species favour. On a longer pre-LGM Pleistocene timescale, Hodgson et al. (2005[9]) have described changes in lake diatom communities in some continental East Antarctic lakes proposed to have survived intact throughout the LGM period. They provide evidence that sub-Antarctic diatom taxa present during the last interglacial period were lost from the community as the LGM approached, leaving only continental taxa, and that the sub-Antarctic taxa have not yet returned to the lakes post LGM.
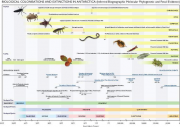
Liquid water and ice-free refugia during ice ages have meant long availability of habitat for some of the biota (e.g. mites, springtails, chironomids), even extending back to the Gondwana era (Allegrucci et al., 2006[10]; Hodgson et al., 2006a[11]; Convey et al., 2008[3]) (Figure 3.29). Nevertheless, the expansion and contraction of the Antarctic ice sheets has undoubtedly led to the local extinction of biological communities on the Antarctic continent during glacial periods (Hodgson et al., 2006a[11]). Subsequent interglacial re-colonisation and the resulting present-day biodiversity is then a result of whether the species were vicariant (surviving the glacial maxima in refugia, possibly also requiring them to take advantage of diachronous extension and retreat of ice in particular areas, then recolonising deglaciated areas), arrived through post-glacial dispersal from lower latitude islands and continents that remained ice free (Pugh et al., 2002[12]), or are present through a combination of both mechanisms. Evidence can be found to support both vicariance (Marshall and Coetzee, 2000[13]; Stevens and Hogg, 2003[7]; Hodgson et al., 2005[9]; Allegrucci et al., 2006[10]; Hodgson et al., 2006a[11]; Stevens et al., 2006[5]; Stevens and Hogg, 2006[14]) and dispersal (Hodgson et al., 2006a[11]) for a variety of different species, and is based on the level of cosmopolitanism (dispersal model) or endemism (vicariance model) (Gibson and Bayly, 2007[15]), on direct palaeolimnological evidence, or, most recently, on molecular phylogenetic and evolutionary studies (Marshall and Convey, 2004[16]; Allegrucci et al., 2006[10]; Stevens et al., 2006[5]; Stevens and Hogg, 2006[14]).
On the oceanic islands, the biotas must have originally arrived via long-distance over-ocean dispersal, with vicariance and terrestrial dispersal playing subsequent roles in shaping the biodiversity across glacial cycles (Marshall and Convey, 2004[16]; Stevens et al., 2006[5]). Species on Southern Ocean islands show conventional island biogeographic relationships, with variance in indigenous species richness explained by factors including area, mean surface air temperature, and age and distance from continental land masses (Marshall and Convey, 2004[16]). For aquatic species, at least for some groups such as the diatoms, diversity is controlled by the ‘connectivity’ among habitats with the more isolated regions developing greater degrees of endemism (Vyverman et al., 2007[17]; Verleyen et al., 2009[18]).
Changing species distributions, abundance and biodiversity
Through the Holocene the changing environmental conditions described in Regional patterns of holocene climate change in Antarctica have caused marked changes in species distributions particularly for those species that have well defined ecological ranges. This is best recorded in palaeolimnological studies where preserved morphological and geochemical fossils provide a detailed record of changing species compositions in response to changes in lake water chemistry and other environmental variables at many sites around the continent.
The historical record of changing terrestrial species distributions is more sparse. Much of our knowledge is based on changes that have been recently been observed in the Antarctic Peninsula region where increasing temperatures in the last 50 years have resulted in the local expansion of population ranges of a number of plant and animal species, and the establishment (albeit with human assistance) of new species that appear not to have survived on the continent before (Frenot et al., 2005[19]; Barnes et al., 2006[20]).
The growth and life cycle patterns of many invertebrates and plants are fundamentally dependent on regional temperature regimes and their linkage with patterns of water availability. Distinct patterns in sexual reproduction are evident across the Antarctic flora and are most likely a function of temperature variation. In addition, phenology (the study of periodic plant and animal life cycle events and how these are influenced by seasonal and interannual variations in climate) of flowering plants is cued to seasonality in the light regime. In regions supporting angiosperms, wind is assumed to play a major role in the pollination ecology of grasses and sedges, resulting in cross-pollination. The lack of pollinators in the native fauna, combined with high reproductive outputs in non-wind pollinated species implies a high reliance on self-pollination.
The Antarctic biota shows high development of ecophysiological adaptations relating to cold and desiccation tolerance, and displays an array of traits to facilitate survival of these conditions. While patterns in absolute low temperatures are clearly influential in determining survival, perhaps more influential are the patterns of sub-lethal environmental stresses experienced and the freeze-thaw regime, with repeated freeze-thaw events being more damaging than a sustained freeze event. How these patterns change in the future will be an issue of major importance to ecosystems.
In contrast to many Antarctic marine organisms, the terrestrial biota often has a wide environmental tolerance. It includes some of the most robust life forms on Earth, the cyanobacteria, which can survive extremes of low temperature, water availability, light and high UV radiation (Hodgson et al., 2004b[21]). These are particularly abundant in extreme habitats, such as parts of the Transantarctic Mountains, where they have no or few competitors. Other groups, however, do have well defined ranges within which they can survive.
It is already well known that Antarctic terrestrial biota possess very effective stress tolerance strategies, in addition to considerable response flexibility. The exceptionally wide degree of environmental variability experienced in many Antarctic terrestrial habitats, on a range of timescales between hours and years, means that predicted levels of change in environmental variables (particularly temperature and water availability) are often small relative to the range already experienced. Given the absence of colonisation by more effective competitors, predicted and observed levels of climate change may be expected to generate positive responses from resident biota of the maritime and continental Antarctic. The picture is likely to be far more complex on the different subantarctic islands, and many already host (different) alien invasive taxa, some of which already have considerable impacts on native biota (Frenot et al., 2005[19]).
References
- ↑ Hodgson, D.A., Doran, P.T., Roberts, D. and McMinn, A. 2004a. Paleolimnological studies from the Antarctic and subantarctic islands. In: Pienitz R, Douglas MSV, Smol JP (eds) Developments in Palaoenvironmental Research. Long-term Environmental Change in Arctic and Antarctic Lakes, 8, Springer, Dordrecht, 419-474.
- ↑ Convey, P. and Stevens, M.I. 2007. Antarctic Biodiversity, Science, 317, 1877-1878.
- ↑ 3.0 3.1 Convey, P., Gibson, J.A.E., Hillenbrand, C-D., Hodgson, D.A., Pugh, P.J.A., Smellie, J.L. and Stevens, M.I. 2008. Antarctic terrestrial life - challenging the history of the frozen continent? Biological Reviews, 83, 103-117.
- ↑ 4.0 4.1 Pugh, P.J.A. and Convey, P. 2008. Surviving out in the cold: Antarctic endemic invertebrates and their refugia, Journal of Biogeography, 35, 2176-2186.
- ↑ 5.0 5.1 5.2 5.3 5.4 Stevens, M.I., Greenslade, P., Hogg, I.D. and Sunnucks, P. 2006. Southern hemisphere springtails: could any have survived glaciation of Antarctica?, Mol. Biol. Evol., 23, 874-882.
- ↑ Bergstrom, D.M. and Chown, S.L. 1999. Life at the front: history, ecology and changes on southern ocean islands, Trends in Ecology and Evolution, 14, 427-477.
- ↑ 7.0 7.1 Stevens, M.I. and Hogg, I.D. 2003. Long-term isolation and recent range expansion revealed for the endemic springtail Gomphiocephalus hodgsoni from southern Victoria Land, Antarctica, Molecular Ecology, 12, 2357-2369.
- ↑ 8.0 8.1 Hodgson, D.A. and Convey, P. 2005. A 7000-year record of oribatid mite communities on a maritime-Antarctic island: responses to climate change, Arctic Antarctic and Alpine Research, 37, 239-245.
- ↑ 9.0 9.1 Hodgson, D.A., Verleyen, E., Sabbe, K., Squier, A.H., Keely, B.J., Leng, M.J., Saunders, K.M. and Vyverman, W. 2005. Late Quaternary climate-driven environmental change in the Larsemann Hills, East Antarctica, multi-proxy evidence from a lake sediment core, Quaternary Research, 64, 83-99.
- ↑ 10.0 10.1 10.2 Allegrucci, G., Carchini, G., Todiscso, V., Convey, P. and Sbordoni, V. 2006. A molecular phylogeny of Antarctic Chironomidae and its implications for biogeographical history. Polar Biology, 29, 320-326.
- ↑ 11.0 11.1 11.2 11.3 Hodgson, D.A., Verleyen, E., Squier, A.H., Sabbe, K., Keely, B.J., Saunders, K.M. and Vyverman, W. 2006a. Interglacial environments of coastal east Antarctica: comparison of MIS 1 (Holocene) and MIS 5e (Last Interglacial) lake-sediment records, Quaternary Science Reviews, 25, 179-197.
- ↑ Pugh, P.J.A., Dartnall, H.J.G. and McInnes, S.J. 2002. The non-marine crustacea of Antarctica and the islands of the Southern Ocean: biodiversity and biogeography. Journal of Natural History 36:1047-1103.
- ↑ Marshall, D.J. and Coetzee, L. 2000. Historical biogeography and ecology of a continental Antarctic mite genus, Maudheimia (Acari, Oribatida): evidence for a Gondwanan origin and Pliocene-Pleistocene speciation, Zoological Journal of the Linnean Society, 129, 111-128.
- ↑ 14.0 14.1 Stevens, M.I. and Hogg, I.D. 2006. The molecular ecology of Antarctic terrestrial and limnetic invertebrates and microbes. In: Bergstrom DM, Convey P, Huiskes AHL (eds) Trends in Antarctic Terrestrial and Limnetic Ecosystems: Antarctica as a global indicator. Springer, Dordrecht, 177-192.
- ↑ Gibson, J.A.E. and Bayly, I.A.E. 2007. New insights into the origins of crustaceans of Antarctic lakes, Antarctic Science, 19, 157-164.
- ↑ 16.0 16.1 16.2 Marshall, D.J. and Convey, P. 2004. Latitudinal variation in habitat specificity of ameronothroid mites, Experimental and Applied Acarology, 34, 21-35.
- ↑ Vyverman, W., Verleyen, E., Sabbe, K., Vanhoutte, K., Sterken, M., Hodgson, D.A., Mann, D.G., Juggins, S., Van De Vijver, B., Jones, V.J., Flower, R., Roberts, D., Chepurnov, V.A., Kilroy, C., Vanormelingen, P. and De Wever, A. 2007. Historical processes constrain patterns in global diatom diversity, Ecology, 88, 1924-1931.
- ↑ Verleyen, E., Vyverman, W., Sterken, M., Hodgson, D.A., De Wever, A., Juggins, S., Van De Vijver, B., Jones, V.J., Vanormelingen, P., Roberts, D., Flower, R., Kilroy, C., Souffreau, C. and Sabbe, K. 2009. The importance of dispersal related and local factors in shaping the taxonomic structure of diatom metacommunities, Oikos, 118, 1239-1249.
- ↑ 19.0 19.1 Frenot, Y., Chown, S.L., Whinam, J., Selkirk, P., Convey, P., Skotnicki, M. and Bergstrom, D. 2005 Biological invasions in the Antarctic: extent, impacts and implications, Biological Reviews, 80, 45-72.
- ↑ Barnes, D.K., Hodgson, D.A., Convey, P., Allen, C.S. and Clarke, A.C. 2006. Incursion and excursion of Antarctic biota: past, present and future, Global Ecol Biogeogr, 15, 121-142.
- ↑ Hodgson, D.A., Vyverman, W., Verleyen, E., Sabbe, K., Leavitt, P.R., Taton, A., Squier, A.H. and Keely, B.J. 2004b. Environmental factors influencing the pigment composition of in situ benthic microbial communities in east Antarctic lakes, Aquatic Microbial Ecology, 37, 247-263.