Satellite observations of the Southern Ocean
- This page is part of the topic Observations, data accuracy and tools
Satellites underpin a vast amount of modern oceanography, and their utility is nowhere greater than in data-poor regions of the Southern Ocean. They include, for example: - altimeters, scatterometers, infra-red and microwave sensors for sea-surface temperature and ice extent and visible-wavelength radiometers for ocean colour. Ensuring their continuity is of paramount importance.
Contents
Sea Surface Temperature
Sea surface temperature is an important geophysical parameter, providing the boundary condition used in the estimation of heat flux at the air-sea interface. On the global scale this is important for climate modelling, study of the Earth's heat balance, and obtaining insight into atmospheric and oceanic circulation patterns and anomalies (such as El Niño). On the mesoscale, SST can be used to study ocean structure, such as eddies, fronts and upwellings and to assess biological productivity. In the remote Southern Ocean where in-situ measurements are particularly sparse, satellite observations of SST are key inputs to studies of physical and biological aspects of oceanography.
Satellite-derived SST is one of the longest duration continuous remote sensing datasets. Observations began in November 1981 using data collected by the AVHRR instruments on the NOAA polar orbiting operational meteorologicall satellites. This series is still operational and plans are in place to continue availability of AVHRR data from the European MetOp and U.S. National Polar-orbiting Operational Environmental Satellite System (NPOESS) series.
The direct broadcast capability of instruments such as the AVHRR adds value for real-time support to scientific cruises in the Southern Ocean.
Improved and consistent processing of AVHRR data has resulted in the Pathfinder dataset (http://podaac.jpl.nasa.gov/PRODUCTS/p216.html), which provides global datasets from 1985 to current at 4 km pixel spacing with improved ice and land masks. This has provided improved SST data sets of the Southern Ocean, which is a very cloudy region where detection of cloud and ice has been a problem in the past.
Other processing efforts include the Global High-Resolution Sea Surface Temperature (GHRSST) project (http://www.ghrsst-pp.org/), which aims to provide a new generation of global multi-sensor high-resolution (<10 km) SST products to the operational oceanographic, meteorological, climate and general scientific community.
Satellite SST data are also available from a range of other satellites, including the European Space Agency Along Track Scanning Radiometer (ATSR) instruments and more recently the MODIS instruments on the NASA Terra and Aqua satellites (Figure 2.14).
Of particular application to the study of climate change are the ATSR instruments onboard the European Space Agency ERS and Envisat satellites. Operating since July 1991, ATSR is now available as consistently processed dataset with a target SST accuracy of 0.3ºC (Corlett et al., 2006[1]). This series of accurate space-based observations of SST is to be extended as part of the European Space Agency Sentinel series, onboard Sentinel-3 and currently planned for launch in 2012.
In addition to SST data derived from thermal infrared measurements, observations are also made using passive microwave measurements. Accuracy and resolution is poorer for SST derived from passive microwave measurements. The advantage gained with passive microwave is that radiation at these longer wavelengths is largely unaffected by clouds, and generally easier to correct for atmospheric effects. Instruments that have been used include the Scanning Multichannel Microwave Radiometer (SMMR) carried on Nimbus-7 and Seasat satellites, the Tropical Rainfall Measuring Mission (TRMM) Microwave Imager (TMI) and data from the AMSR instrument on the NASA EOS Aqua satellite.
Altimetry
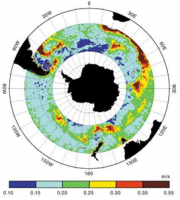
Satellite altimeters provide data that are helpful for understanding both the ocean circulation (Gille et al., 2000[3]; Hughes et al., 2001) and the geophysical characteristics of the sea floor (Sandwell and Smith, 1997[4]) (Figure 2.15). Satellite altimeters provide long-term observations of mesoscale circulation patterns in the Southern Ocean and the variability of features such as the ACC. Satellite altimetry also provides information that is of use in mapping sea surface wind speeds and significant wave heights.
In the polar oceans, sea ice and its associated snow cover is a major regulator of the heat, mass and momentum between the atmosphere and the ocean. Although ice extent and concentration are routinely measured from space, sea ice and snow thickness, particularly in the Antarctic, are not well measured and are highly uncertain. Methods for estimating sea ice thickness in the Arctic using satellite altimetry may have application for measuring Antarctic sea ice thickness, despite the difficulty of determining the smaller freeboard measurements of dominant first year ice in the Antarctic (Giles et al., 2006[5]).
Radar altimeters are non-imaging radar sensors, which use the ranging capability of radar to measure the surface topographic profile parallel to the satellite track. They provide precise measurements of a satellite’s height above the ocean and, if appropriately designed, over land/ice surfaces, by measuring the time interval between the transmission and reception of very short electromagnetic pulses.
To date, most spaceborne radar altimeters have been wide-beam (pulse-limited) systems operating from low Earth orbits. Radar altimetry data have been collected from a variety of instruments including Seasat (1978), Geosat (1985-1989), ERS-1 (1991-1996), Topex-Poseidon (since 1992), ERS-2 (since 1995), Jason-1 (since 2001) and Envisat (since 2002). The future availability of satellite altimetry observations is assured with new missions such as Jason-2 and the ESA Sentinel satellites. ESA’s CryoSat-2 mission will also provide new data from the SIRAL instrument specifically designed to deliver higher resolution measurements for ice sheet and sea ice observations.
In addition to radar altimetry, the first satellite laser altimeter was launched in 2003 onboard the Ice, Cloud, and land Elevation Satellite (ICESat) satellite. The Geoscience Laser Altimeter System (GLAS) is the sole instrument on the ICESat (Figure 2.16). The main objective of the ICESat mission is to measure ice sheet elevations and changes in elevation through time. Secondary objectives include sea ice thickness, measurement of cloud and aerosol height profiles, land elevation and vegetation cover (Schutz et al., 2005[6]). NASA’s ICESat-2 mission will continue the legacy of ICESat from around 2014.
Ocean colour
Satellite ocean colour sensors provide data on the concentration of chlorophyll in the ocean surface waters. Variations in ocean chlorophyll concentration contribute information on the biology of the Southern Ocean and are a key input for modeling of the South Ocean ecosystem. In a region characterised by high-nutrient, low-chlorophyll status, ocean colour satellite imagery has greatly enhanced our understanding of the true spatial and temporal extent of phytoplankton blooms (Figure 2.17) and has revealed that chlorophyll-a (chl-a) biomass can be particularly high in regions such as the Scotia and Weddell Seas (E.J. Murphy et al., 2006[7]). Combined with other observations, chlorophyll measurements help to provide an understanding of how ocean productivity changes with weather, oceanographic variations such as the El Niño/South Oscillation, and other fluctuations in ocean temperature – work that could provide hints as to how future climatic change could affect ocean productivity (Behrenfeld et al., 2006[8]).
Parts of the Southern Ocean ecosystem have also been highly perturbed as a result of harvesting over the last two centuries, and significant ecological changes have also occurred in response to rapid regional warming during the second half of the twentieth century. This combination of historical perturbation and rapid regional change suggests that the Scotia Sea ecosystem is likely to show significant change over the next two to three decades, which may result in major ecological shifts. Satellite measurements of chlorophyll will help obtain a comprehensive understanding of the evolution of the Antarctic marine ecosystem.
The accuracy of chlorophyll measurements varies from region to region globally. In the Southern Ocean it has been observed (Holm-Hansen et al., 2004[9]) that satellite data underestimate chlorophyll values recorded in situ at high chlorophyll concentrations, and slightly over-estimate the shipboard data at lower chlorophyll concentrations.
Data suitable for measurement of chlorophyll concentration began in 1979 with the launch of the Coastal Zone Colour Scanner (CZCS) onboard the Nimbus-7 satellite. Intermittent collection of data continued until 1986. However, it was not until 1997 that global ocean colour observations resumed with the launch of the SeaWiFS (Sea-Viewing Wide Field-of-View Sensor) instrument onboard the Orbview-2 satellite. SeaWiFS has now collected data for more than a decade, but continuity of ocean colour data continues concurrently with the MODIS instruments on the Terra and Aqua satellites and the MERIS instrument on the Envisat satellite. Future plans for the continuity of satellite chlorophyll measurements include instruments on the ESA Sentinel-2 satellite and the NOAA Polar-orbiting Operational Environmental Satellite System (NPOESS) series.
Scatterometer data
Radar scatterometer instruments have been designed principally to capture the near-surface wind field over the oceans. By assuming that the energy transmitted back to the radar from the ocean is dependent only upon that component of sea surface roughness that is a product of the frictional interaction of wind on the surface, a model may be used to relate this roughness, through the radar backscatter coefficient, to wind speed and direction. This is possible because the roughness is anisotropic, with crests and troughs generally orthogonal to the wind direction.
The primary use of scatterometer data in southern high latitudes is as data to be assimilated into numerical weather prediction (NWP) models. For example, Andrews and Bell (1998[10]) showed a marked reduction in rms errors of UK Met Office forecasts over the Southern Ocean that assimilated scatterometer winds. However, the spatial resolution of scatterometer data — 25 km for the instrument on the ERS-1 satellite — means that it can also be used for case studies. It has been utilised to study both mesoscale and synoptic-scale weather systems around Antarctica (Marshall and Turner, 1997a[11], 1999[12]) and coastal katabatic winds off the Ross Ice Shelf (Marshall and Turner, 1997b[13]).
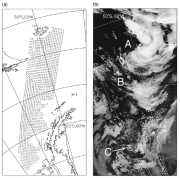
Figure 2.18, taken from Marshall and Turner (1999[12]) demonstrates the type of information that scatterometer data can provide about weather systems over the Southern Ocean. Feature A is a mesocyclone and it can be observed in the scatterometer data: wind speeds are greater on the equatorward side and weaker on the poleward side of the centre than the background flow, and the wind field shows troughing in an equatorward direction. Feature B is a trough that is not apparent in the cloud imagery: the scatterometer data indicate the marked wind shear associated with this trough, with the wind direction changing by 135° within the resolution of the data. Finally, feature C is a dissipating synoptic-scale system located close to the Antarctic Peninsula. The scatterometer winds reveal a weak but closed surface circulation.
Scatterometer data can also provide useful information from ice-covered surfaces (Long et al., 2001[14]): the return signal is dependent on the roughness and the dielectric properties (a measure of the ability of a medium to resist the formation of an electric field within it) of the surface and near-surface. Despite the relatively poor spatial resolution, useful information on the thermodynamic state, distribution and dynamics of sea ice at a regional scale can be tracked easily because of the frequent repeat coverage at polar latitudes (1-2 days). Similarly, over terrestrial ice sheets large-scale patterns of seasonal melt can be observed.
Synthetic Aperture Radar
Imaging Synthetic Aperture Radar (SAR) systems provide imagery of the ocean and land surfaces by recording the reflected signals of emitted microwave radiation (Figure 2.19). The active nature of these imaging instruments means data can be obtained throughout the year, day or night and regardless of cloud and weather conditions. Whilst initially difficult to interpret, they are useful in resolving features of sea ice, land ice and snow, and the oceans.
SAR imagery also has wide application to oceanographic studies. Imagery provides information about the interactions at the sea ice edge and the resolution of currents, frontal boundaries and internal waves.
Available SAR imaging satellites began in 1991 with the launch of the European ERS-1 satellite. Since then, ERS-2, Envisat and Radarsat satellites have provided continuity of C-band SAR imagery. In 1997, Radarsat even made an unprecedented in-orbit manoeuvre to allow the entire Antarctic continent to be imaged including areas south of 80º S, which are not normally viewable. This resulted in the RAMP dataset (http://bprc.osu.edu/rsl/radarsat/radarsat.html, which has subsequently been updated in 2000 to provide the MAMM (Modified Antarctic Mapping Mission) dataset, which includes repeat images of glaciers in order to determine velocity. Recently, other SAR systems including different wavelength radars have been launched, including Radarsat-2, the Japanese ALOS, TerraSAR-X and COSMO-SkyMed systems. In the future there are plans for continuity of the European SAR systems from the ESA Sentinel series, a Radarsat constellation, a planned addition to the TerraSAR system called Tandem-X that will deliver detailed elevation data and even discussion of a P-band radar system that may allow imaging of internal ice sheet structure.
References
- ↑ Corlett, G.K., Barton, I.J., Donlan, C.J., Edwards, M.C., Good, S.A., Horrocks, L.A., Llewellyn-Jones, D.T., Merchant, C.J., Minnett, P.J., Nightingale, T.J., Noyes, E.J., O'carroll, A.G., Remedios, J.J., Robinson, I.S., Saunders, R.W. and Watts, J.G. 2006. The accuracy of SST retrievals from AATSR: An initial assessment through geophysical validation against in situ radiometers, buoys and other SST data sets, Advances in Space Research, 37 (4), 764-769.
- ↑ Gille, S.T. and Sandwell, D.T. 2001. Gravity, Bathymetry, and Mesoscale Ocean Circulation from Altimetry, AVISO newsletter, 8, September.
- ↑ Gille, S.T., Yale, M.M. and Sandwell, D.T. 2000. Correlation of mesoscale ocean variability with seafloor roughness from satellite altimetry, Geophys. Res. Lett., 27, 1251-1254.
- ↑ Sandwell, D.T. and Smith, W.H.F. 1997. Marine gravity anomaly from Geosat and ERS-1 satellite altimetry, J. Geophys. Res., 102, 10,039-10,054.
- ↑ Giles, K.A., Laxon, S.W. and Worby, T. 2006. Validating Satellite Radar Altimetry Estimates of Antarctic sea ice Thickness Using the ASPeCt Data set, Proceedings of 2006 American Geophysical Union Fall Meeting.
- ↑ Schutz, B.E., Zwally, H.J., Shuman, C.A., Hancock, D. and Dimarzio, J.P. 2005. Overview of the ICESat Mission, Geophys. Res. Lett., 32, L21S01, doi:10.1029/2005GL024009.
- ↑ Murphy, E.J., Watkins, J.L., Trathan, P.N., Reid, K., Meredith, M.P., Thorpe, S.E., Johnston, N.M., Clarke, A., Tarling, G.A., Collins, M.A., Forcada, J., Shreeve, R.S., Atkinson, A., Korb, R., Whitehouse, M.J., Ward, P., Rodhouse, P.G., Enderlein, P., Hirst, A.G., Martin, A.R., Hill, S.L., Staniland, I.J., Pond, D.W., Briggs, D.R., Cunningham, N.J. and Fleming, A.H. 2006. Spatial and temporal operation of the Scotia Sea ecosystem: a review of large-scale links in a krill centred food web, Philosophical Transactions of the Royal Society B: Biological Sciences, 362, (1477), DOI: 10.1098/rstb.2006.1957.
- ↑ Behrenfeld, M., O’Malley, R., Siegel, D., McClain, C., Sarmiento, J., Feldman, G., Milligan, A., Falkowski, P., Letelier, R. and Boss, E. 2006. Climate-driven trends in contemporary ocean productivity, Nature, 444, 752-755.
- ↑ Holm-Hansen, O., Kahru, M., Hewes, C.D., Kawaguchi, S., Kameda, T., Sushin, V.A., Krasovski, I., Priddle, J., Korb, R., Hewitt, R.P. and Mitchell, B.G. 2004. Temporal and spatial distribution of chlorophyll-a in surface waters of the Scotia Sea as determined by both shipboard measurements and satellite data, Deep-Sea Research Part II—Topical Studies in Oceanography, 51 (2004) (12-13), 1323-1332.
- ↑ Andrews, P.L. and Bell, R.S. 1998. Optimizing the United Kingdom Meteorological Office data assimilation for ERS-1 scatterometer winds. Monthly Weather Review, 126, 736-746.
- ↑ Marshall, G.J. and Turner, J. 1997a. Surface wind fields of Antarctic mesocyclones derived from ERS-1 scatterometer data, Journal of Geophysical Research, 102, 13907-13921.
- ↑ 12.0 12.1 12.2 Marshall, G.J. and Turner, J. 1999. Synoptic-scale weather systems observed during the FROST project via scatterometer winds, Weather and Forecasting, 14, 867 877.
- ↑ Marshall, G.J. and Turner, J. 1997b. Katabatic wind propagation over the western Ross Sea observed using ERS-1 scatterometer data, Antarctic Science, 9, 221-226.
- ↑ Long, D.G., Drinkwater, M.R., Holt, B., Saatchi, S. and Bertoia, C. 2001. Global ice and land climate studies using scatterometer image data, EOS, Transactions of the American Geophysical Union, 82, 503 (see also http://www.agu.org/eos_elec/010126e.html).