ENSO links and teleconnections to vertebrate life histories and population
- This page is part of the topic Marine biology in the instrumental period
Biological impacts with consequences for upper trophic levels in the southwest Atlantic region occur at the same time as the rapid warming observed to the west of the Antarctic Peninsula, in the Amundsen Sea and across the Bellingshausen Sea, and to the east of the Peninsula across the northwestern Weddell Sea, where the sea ice season has decreased (Parkinson, 2004[1]). Food webs in these regions are dominated by euphausids, and in particular by Antarctic krill, Euphausia superba, which provide ecosystem structure and function, supporting the energetic demands of abundant predator populations (Croxall et al., 1988[2]) and also a commercial fishery. As shown above, the relative density of krill across the region has shown a significant negative trend over the last 30 years, during which summer mean krill density correleated positively with the duration of sea ice the previous winter (Atkinson et al., 2004[3]).
The strong connections between sea ice and ENSO variability across the southwest Atlantic result in correlations between ENSO variation and krill recruitment and abundance; quasi-cyclic sea ice variation is related to cycles in krill population (Fraser and Hofmann, 2003[4]; Quetin and Ross, 2003[5]). Recent studies have shown how ENSO related fluctuations in SST and winter sea ice extent affect the recruitment and dispersal of Antarctic krill. Delayed effects of sea ice conditions on abundance relate to the production, survival and development of the larval and juvenile krill. Same-year effects of spring and summer temperatures probably reflect a distribution and dispersal effect across the Scotia Sea and the degree of influence of cooler polar waters in northern regions around South Georgia (Murphy et al., 1998[6], 2007[7]).
These effects cascade, bottom-up, to upper trophic levels. With few exceptions, periods of reduced predator breeding performance in this region are the result of low prey availability rather than direct local weather or oceanic effects (Croxall et al., 1988[2]; Fraser and Hofmann, 2003[4]; Forcada et al., 2005[8], 2006[9]; Trathan et al., 2006[10]; Hinke et al., 2007[11]; Murphy et al., 2007[7]).
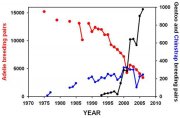
For Adélie penguins breeding on the western Antarctic Peninsula, changes in sea ice extent during the breeding season covary positively with krill abundance and negatively with penguin foraging effort (Fraser and Hofmann, 2003[4]). In the South Shetland Islands, krill recruitment covaries positively with Adélie penguin recruitment, which has declined dramatically (Hinke et al., 2007[11]). The population trends of Adélie and Chinstrap penguins appear to be affected by a winter krill deficit. This deficit also affects the Gentoo penguins, but their population trends are positive (Figure 4.48, Ducklow et al., 2007[12]). Similar results on population trends at the South Orkneys suggest the loss of buffering against the changing sea ice environment by the more abundant and ice-dependent Chinstrap and Adélie penguins, and positive population consequences through habitat improvement for the less ice-related Gentoo penguin (Forcada et al., 2006[9]).
Other krill dependent predators have shown comparable responses to the propagation of ENSO variability. Breeding outputs of Gentoo penguins and Antarctic fur seals at Bird Island, South Georgia (Forcada et al., 2005[8]; Trathan et al., 2006[10]) and southern right whales (Eubaleana australis), which feed in the waters around South Georgia (Leaper et al., 2006[13]), correlate negatively with SST anomalies. Low chick and pup production and survival in penguins and fur seals result from a reduced food supply, usually of krill and krill-dependent fish species, such as mackerel icefish.
Signals of climate change related to ENSO variability are also observed elsewhere in the Southern Ocean. However, the consequences for ecosystem fluctuation are less well understood. In the Indian Ocean, and in particular in regions of the Antarctic continent, long-term reductions in sea ice extent have occurred in parallel with reductions of the Southern Oscillation Index (and an opposite trend in ENSO) (Barbraud and Weimerskirch, 2006[14]). Long-term increases in sea ice season duration have possibly reduced the quantity and accessibility of the food supplies available in early spring for upper trophic levels. This would partly explain the delays in phenology observed in a guild of seabird species (Barbraud and Weimerskirch, 2006[14]). While little is known about the marine ecosystem structure and food web interactions, there have been major fluctuations in the late 1970s suggesting a regime shift with repercussions for lower trophic levels (Hunt et al., 2001[15]), but also for upper trophic levels, and in particular Emperor penguins, which suffered a major population decline (Weimerskirch et al., 2003[16]). Long-term environmental effects in breeding success were further explored in long-term data sets of seabirds, including southern fulmars, snow petrels and emperor penguins in Terre Adélie. The observed population fluctuation in these species had a periodicity of 3-5 years, consistent with that in sea ice anomalies and the SOI (and hence ENSO). The observed cyclical patterns also indicated significant change since 1980, consistent with a regime shift, although these populations have remained stable since then.
In the Antarctic sector of the Pacific Ocean, and in particular the Ross Sea sector, trends in surface temperatures are less apparently correlated with ENSO, although there has been a decrease in the SOI. The impacts may be less evident or may even be the reverse of those observed in the Atlantic and the Indian Oceans. Annual estimates of breeding population size for Adélie penguin colonies on Ross Island, in the Ross Sea, show significant inter-annual variability, consistent with ENSO and the propagation of its variability through the ACW (Wilson et al., 2001[17]; Ainley et al., 2005[18]). Lagged correlations with a sea ice index and the SOI (ENSO) suggest demographic cycles, with reverse population trends to those observed in the Southwest Atlantic. However the consequences for Adélie penguins changed between the 1970s and the 1980s, with a concurrent increase in population trend and in the sea ice polynya providing improved penguin habitat. Like the Emperor Penguins in Terre Adélie, in the Indian Ocean, populations of Weddell seals at McMurdo Sound, in the Ross Sea, declined in the early 1970s, to become stable later on (Ainley et al., 2005[18]). This suggests that ENSO impacts have not been so obvious in the Pacific sector as in the Atlantic Ocean and Indian Ocean sectors of the Southern Ocean. Based on long-term studies, Dayton (1989[19]) and Barry and Dayton (1988[20]) speculated about ENSO-related hydrodynamic processes supporting the explosive growth of fast growing sponges in McMurdo Sound with significant consequences for the entire benthic assemblage.
References
- ↑ Parkinson, C.L. 2004. Southern Ocean sea ice and its wider linkages: insights revealed from models and observations, Antarctic Science, 16, 387-400.
- ↑ 2.0 2.1 Croxall, J.P., McCann, T.S., Prince, P.A. and Rothery, P. 1988. Reproductive performance of seabirds and seals at South Georgia and Signy Island, South Orkney Islands, 1976-1987: implications for Southerrn Ocean monitoring studies. In Antarctic Ocean resources variability (ed. D Sahrhage), 261-285. Berlin, Germany: Springer.
- ↑ Atkinson, A., Pakhomov, E., Rothery, P., Siegel, V. 2004. Long-term decline in krill stocks and increase in salps within the Southern Ocean, Nature, 432 (7013), 100-103.
- ↑ 4.0 4.1 4.2 Fraser, W.R. and Hofmann, E.E. 2003. A predator’s perspective on causal links between climate change, physical forcing and ecosystem response, Marine Ecology Progress Series, 265, 1-15.
- ↑ Quetin, L.B. and Ross, R.M. 2003. Episodic recruitment in Antarctic krill Euphausia superba in the Palmer LTER study region, Marine Ecology Progress Series, 259, 185-200.
- ↑ Murphy, E.J., Watkins, J.L., Reid, K., Trathan, P.N., Everson, I., Croxall, J.P., Priddle, J., Brandon, M.A., Brierley, A.S. and Hofmann, E. 1998. Interannual variability of the South Georgia marine ecosystem: biological and physical sources of variation in the abundance of krill, Fisheries Oceanography, 7, 381-390.
- ↑ 7.0 7.1 Murphy, E.J., Trathan, P.N., Watkins, J.L., Reid, K., Meredith, M.P., Forcada, J., Thorpe, S.E., Jonston, N.M. and Rothery, P. 2007. Climatically driven fluctuations in Southern Ocean ecosystems, Proceedings of the Royal Society B, doi:10.1098/rspb.2007.1180.
- ↑ 8.0 8.1 Forcada, J., Trathan, P.N., Reid, K. and Murphy E.J. 2005. The effects of global climate variability in pup production of Antarctic fur seals, Ecology, 86, 2408-2417.
- ↑ 9.0 9.1 Forcada, J., Trathan, P.N., Reid, K., Murphy, E.J. and Croxall, J.P. 2006. Contrasting population changes in sympatric penguin species with climate warming, Global Change Biology, 12, 411-423.
- ↑ 10.0 10.1 Trathan, P.N., Murphy, E.J., Forcada, J., Croxall, J.P., Reid, K. and Thorpe, S.E. 2006. Physical forcing in the southwest Atlantic: ecosystem control. In Top predators in marine ecosystems: their role inmonitoring andmanagement (eds I L Boyd, S Wanless and C J Camphuysen), 28-45. Cambridge, UK: Cambridge University Press.
- ↑ 11.0 11.1 Hinke, J.T., Salwicka, K., Trivelpiece, S.G., Watters, G.M. and Trivelpiece, W.Z. 2007. Divergent responses of Pygoscelis penguins reveal a common environmental driver, Oecologia, 153, 845-855.
- ↑ 12.0 12.1 Ducklow, H.W., Baker, K., Martinson, D.G., Quentin, L.B., Ross, R.M., Smith, R.C., Stammerjohn, S.E., Vernet, M. and Fraser, W. 2007. Marine pelagic ecosystems: the West Antarctic Peninsula, Phil. Trans. R. Soc. B, 362, 67-94.
- ↑ Leaper, R., Cooke, J., Trathan, P.N., Reid, K., Rowntree, V. and Payne, R. 2006. Global climate drives southern right whale (Eubalaena australis) population dynamics, Biology Letters, 2, 289-292.
- ↑ 14.0 14.1 Barbraud, C. and Weimerskirch, H. 2006. Antarctic birds breed later in response to climate change, Proceedings of the National Academy of Sciences, 103, 6248-6251.
- ↑ Hunt B.P.V, Pakhomov, E.A. and McQuaid, C.D. 2001. Community structure of mesozooplankton in the Antarctic Polar Frontal Zone in the vicinity of the Prince Edward Islands (Southern Ocean): Small-scale distribution patterns in relation to physical parameters, Deep-Sea Research Part II Topical Studies Oceanography, 49, 3307-3325.
- ↑ Weimerskirch, H., Inchausti, P., Guinet, C. and Barbraud, C. 2003. Trends in bird and seal populations as indicators of a system shift in the Southern Ocean, Antarctic Science, 15, 249-256.
- ↑ Wilson, P.R., Ainley, D.G., Nur, N., Jacobs, S.S., Barton, K.J., Ballard, G. and Comiso, J.C. 2001. Adélie penguin population change in the pacific sector of Antarctica: relation to sea-ice extent and the Antarctic Circumpolar Current, Marine Ecology Progress Series, 213, 301-309.
- ↑ 18.0 18.1 Ainley, D.G., Clarke E.D., Arrigo, K., Fraser, W.R., Kato, A., Barton, K.J. and Wilson, P.R. 2005. Decadal-scale changes in the climate and biota of the Pacific sector of the Southern Ocean, 1950s to the 1990s. Antarctic Science, 17, 171-182.
- ↑ Dayton, P.K. 1989. Interdecadal variation in an Antarctic sponge and its predators from Oceanographic climate shifts, Science, 245, 1484-1486
- ↑ Barry, J.P. and Dayton, P.K. 1988. Current patterns in McMurdo Sound, Antarctica, and their relationship to local biotic communities, Polar Biol., 8, 367-376.