Changes in sea ice extent through the Holocene
- This page is part of the topic The Holocene
Despite the fundamental importance of sea ice to many aspects of Holocene climate (see Sea ice over the last million years), it is a factor that remains poorly constrained in computer simulations of past and future climate change. One of the reasons for this is the paucity of historical and palaeo records of sea ice extent. Routine satellite measurements of sea ice began only in the 1970s, and the strong interannual variability in these short instrumental records makes it difficult to isolate long-term changes in sea ice cover (Zwally et al., 2002a[1]). Whaling records and early sea ice charts do suggest that Antarctic sea ice cover has undergone a dramatic decline during the 20th century (de la Mare, 1997[2]), although the quality and interpretation of these early historical records is debated. In order to improve the incorporation of sea ice into computer models of future climate change it will be essential to use proxy records to represent how Antarctic sea ice has changed in the past.
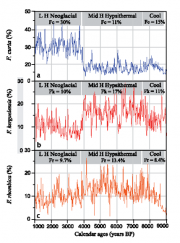
Much of our current understanding of Holocene sea ice changes around Antarctica comes from a few marine sediment cores in which the diatom microfossils are used as a proxy for past sea ice coverage over the core site (Hodell et al., 2001[4]), although these have yet to be calibrated with the instrumental record. Instead, qualitative reconstructions of the presence or absence of sea ice at a core site can be made by measuring changes in the abundance of those diatom species that are associated either with the presence of winter sea ice or with the presence of summer sea ice. Quantitative reconstructions of changes in the duration of sea ice cover at particular sites can be made by identifying changes in the relative abundances of a number of diatom species (Crosta et al., 1998a[5]; Crosta et al., 1998b[6]), see Figure 3.23 from Crosta et al. (2007[3]).
Holocene sediment cores from the Southern Ocean, for example off Adélie Land, East Antarctica, generally record reduced sea ice coverage during the early to mid-Holocene (Figure 3.23) (Hodell et al., 2001[4]). This minimum in sea ice cover is broadly coincident with the timing of a Holocene climatic optimum documented in some marine palaeoclimatic records from the Antarctic continent and Southern Ocean (Masson et al., 2000[7]; Domack et al., 2001[8]). Also known as a Hypsithermal, this marine-inferred Holocene climatic optimum seems to have ended at different times around Antarctica. In the Atlantic sector of the Southern Ocean (offshore from Dronning Maud Land and the Weddell Sea) sediment cores show that marine-inferred conditions were warm and ice free at ~50ºS until around 6-5 ka BP (Hodell et al., 2001[4]; Nielsen et al., 2004[9]). There is some leeway in the dating, which in Hodell’s core was determined at 5 ka by extrapolation from sedimentation rates, leaving room for uncertainty. This marine-inferred mid Holocene warm period was followed by a marine Neoglacial cool period that lasted until at least ~2 ka BP, when winter sea ice cover extended out to beyond the sediment core sites at ~50ºS. This was then followed by a late Holocene warming that saw sea ice retreat southward of 50oS in the Atlantic sector (Nielsen et al., 2004[9]), although the evidence for this warming and sea ice retreat is less obvious in a core site at 53oS (Hodell et al., 2001[4]). At present the timing of this marine-inferred mid-Holocene climate optimum is out of phase with some of the ice core and terrestrial records described in Regional patterns of holocene climate change in Antarctica and requires further study.
In the Southern Ocean sector facing Australia (offshore of Adélie Land in East Antarctica) a diatom record from 66oS suggests that the transition to increased sea ice coverage in this marine-inferred Neoglacial cool period occurred at ~4 ka BP, marked by an abrupt threshold change in the relative abundance of F. kerguelensis and F.curta which have particular ecological responses at 0.5°C, and a more gradual change in F. rhombica (Figure 3.23), the latter giving a signal that is in good agreement with model output (Renssen et al., 2005[10]). This lasted until at least ~1 ka BP (the top of the core) (Figure 3.23) (Crosta et al., 2007[3]). As well as these long-term changes in winter sea ice extent, changes in diatom species also suggest that during the colder Neoglacial period both the autumn formation and spring melting of sea ice occurred later in the season than during the Hypsithermal (Crosta et al., 2008[11]). A sediment core record from the eastern Pacific sector of the Southern Ocean (offshore of the western Antarctic Peninsula) also supports a delayed transition at ~3.6 ka BP to cool marine Neoglacial conditions (Domack et al., 2001[8]).
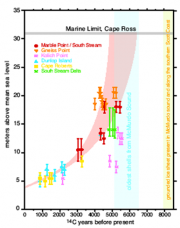
The drivers of this marine-inferred mid-Holocene cooling in ocean temperature and increase in sea ice around Antarctica after ~6 ka BP are still debated. One hypothesis is that cooling of the Southern Ocean during the mid-Holocene may have been a response to the decrease in summer insolation at high latitudes in the Northern Hemisphere (see the insolation curve in Figure 3.16), which may have led to an increase in the strength of the thermohaline circulation (Hodell et al., 2001[4]; Nielsen et al., 2004[9]). However, a numerical model of the influence of seasonal changes in insolation over Antarctica suggests that the observed regional changes in Holocene temperatures and sea ice extent in the Southern Ocean may instead be consistent with forcing by southern summer insolation (Renssen et al., 2005[10]). This model suggests that the temperature and sea ice trends in the Southern Ocean can be explained by the combination of a 1-2 month lag of ocean temperature to local insolation, and the long memory of the Southern Ocean system - which allows local insolation signals from different seasons to be preserved throughout the year. Sea ice also plays an important role in the modelled results by amplifying the effect of the local insolation signal through ice-albedo and ice-insulation feedbacks. This model simulation also suggests that sea ice cover in the early Holocene was more reduced in the western than in the eastern sector of the Southern Ocean, which could account for the regional differences in the timing/length of the Holocene climatic optimum in palaeoclimate records.
As well as recording the seasonal and inter-annual changes in sea ice during the Holocene, diatoms from sediment cores record decadal to millennial-scale cyclic variations in Antarctic sea ice (Nielsen et al., 2004[9]; Crosta et al., 2008[11]). Based on spectral analysis, it has been proposed that many of these high-frequency oscillations (c. 200 yr) in sea ice are consistent with the periodicities in records of solar activity (e.g. ∆14C). Other periodicities in the Holocene diatom records may be associated with internal climate variability related to the global thermohaline circulation, and possibly act to amplify the influence of high-frequency variations in solar activity (Crosta et al., 2008[11]). Verification of the drivers associated with decadal to millennial-scale cyclical changes in sea ice is hampered by the limited resolution and dating control on most marine sediment cores. However, the possibility of developing highly resolved sea ice reconstructions in the future from varved marine sediments or from ice cores (see below) may help to establish the degree to which external (e.g. solar) and internal (e.g. thermohaline circulation) forcings affect the amount of sea ice around Antarctica.
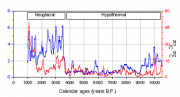
Efforts are currently underway to establish new proxies to help build reliable reconstructions of Antarctic sea ice through the Holocene, and beyond. Promising proxies for the future may come from chemical markers in marine sediment cores from around coastal Antarctica. The presence of the highly branched isoprenoid biomarker known as IP25 in Arctic sea ice and sediments appears to be associated with the Haslea spp. sea ice diatom (Belt et al., 2007[13]). Early studies using sediment cores from the Arctic and Antarctic have found that the sea ice reconstructions produced using IP25 and similar biomarkers are consistent with historical sea ice reports (Massé et al., 2008[14]) and sea ice estimates based on diatom assemblages (Figure 3.24). These biomarkers can be measured rapidly in extremely small sample sizes and have been readily detected in sediments covering the entire Holocene. Although their long-term behaviour in sediments needs to be established further, it appears that these biomarkers will play a valuable role in the development of proxy sea ice records around Antarctica.
The chemistry of Antarctic ice cores may also provide proxies for reconstructing the Holocene history of Antarctic sea ice. Sea salt in ice cores has traditionally been viewed as a proxy for wind transport strength and storminess (Iizuka et al., 2008[15]). However, the observation of increased sea salt in Antarctic ice cores during winter and during glacial periods, as well as a characteristic sulphate depletion in the sea salt aerosol reaching Antarctica, has recently led to the suggestion that sea salt may instead reflect the formation of brine and frost flowers on top of new sea ice (Rankin et al., 2002[16]; Wolff et al., 2003[17]). Ice core records of sea salt appear to respond to sea ice changes over long timescales (e.g. glacial-interglacial), but more quantitative calibration is required (Wolff et al., 2006[18]; Fischer et al., 2007[19]). While sea salt is a promising proxy from a conceptual point of view, it still needs to be shown whether it is sensitive to changes in sea ice production over short timescales of centuries or less. It also appears that the response of this proxy reaches a threshold level when sea ice is very extensive (i.e. during glacial periods).
Another promising sea ice proxy in Antarctic ice cores is methane sulphonic acid (MSA) (Curran et al., 2003[20]). MSA is ultimately derived from marine phytoplankton that thrive in the marginal sea ice zone. At some ice core sites around coastal Antarctica the amount of MSA deposited in Antarctic snow increases following winters of increased sea ice extent (Welch et al., 1993[21]; Curran et al., 2003[20]; Abram et al.. 2007b). However, at other locations MSA variability appears to be more sensitive to wind direction (Fundel et al., 2006[22]; Abram et al., 2007b[23]). This means that site selection and transport processes are essential considerations in using ice core records as sea ice proxies. Post-depositional changes in MSA records at sites with low accumulation rates and over long time periods also mean that the use of this proxy is essentially limited to high accumulation records of the Holocene.
Reconstructions of Antarctic sea ice extent based on proxy data from ice cores have the advantage of providing a regionally averaged view, rather than being sensitive to sea ice conditions over a single (coring) point in the Southern Ocean. Ice core records also have the potential to provide both long-term records of sea ice changes through the Holocene, and very detailed (i.e. annual resolution) reconstructions of sea ice changes during recent centuries. Early comparisons of high-resolution MSA reconstructions of sea ice extent from sites around the Antarctic continent reveal clear regional differences in the timing and speed of Antarctic sea ice decline (and growth) during the 20th century (Curran et al., 2003[20]; Abram et al., 2007a[24]).
References
- ↑ Zwally, H.J, Comiso, J.C., Parkinson, C.L., Cavalieri, D.J. and Gloersen, P. 2002a. Variability of Antarctic sea ice 1979-1998, Journal of Geophysical Research, 10, 3041, doi: 3010.1029/2000JC000733.
- ↑ De La Mare, W.K. 1997. Abrupt mid-twentieth century decline in Antarctic sea-ice extent from whaling records, Nature, 389 (6646), 57-61.
- ↑ 3.0 3.1 3.2 Crosta, X., Debret, M., Denis, D., Courty, M-A. and Ther, O. 2007 Holocene long- and short-term climate changes off Adelie Land, East Antarctica. Geochemistry Geophysics Geosystems, 8, Q11009, doi:11010.11029/12007GC001718.
- ↑ 4.0 4.1 4.2 4.3 4.4 Hodell, D.A., Kanfoush, S.L., Shemesh, A., Crosta, X., Charles, C.D. and Guilderson, T.P. 2001. Abrupt cooling of Antarctic surface waters and sea ice expansion in the South Atlantic sector of the Southern Ocean at 5000 cal yr B.P., Quaternary Research, 56, 191-198.
- ↑ Crosta, X., Pichon, J-J. and Burckle, L.H. 1998a. Application of Modern Analog Technique to marine Antarctic diatoms: Reconstruction of maxiumum sea ice extent at the Last Glacial Maximum, Paleoceanography, 13, 284-297.
- ↑ Crosta, X., Pichon, J.J. and Burckle, L.H. 1998b. Reappraisal of Antarctic seasonal sea-ice at the Last Glacial Maximum, Geophysical Research Letters, 25, 2703-2706.
- ↑ Masson, V., Vimeux, F., Jouzel, J., Morgan, V., Delmotte, M., Ciais, P., Hammer, C., Johnsen, S., Lipenkov, V.Y., Mosley-Thompson, E., Petit, J.R., Steig, E.J., Stievenard, M. and Vaikmae, R. 2000. Holocene climate variability in Antarctica based on 11 ice-core isotope records, Quaternary Research, 54, 348-358.
- ↑ 8.0 8.1 Domack, E., Leventer, A., Dunbar, R.B., Taylor, F., Brachfeld, S., Sjunneskog, C. and Party, O.L.S. 2001. Chronology of the Palmer Deep site, Antarctic Peninsula: a Holocene palaeoenvironmental reference for the circum-Antarctic, The Holocene, 11, 1-9.
- ↑ 9.0 9.1 9.2 9.3 Nielsen, S.H.H., Koc, N. and Crosta, X. 2004. Holocene climate in the Atlantic sector of the Southern Ocean: controlled by insolation or oceanic circulation?, Geology, 32, 317-320.
- ↑ 10.0 10.1 Renssen, H., Goosse, H., Fichefet, T., Masson-Delmotte, V. and Koc, N. 2005. Holocene climate evolution in the high-latitude Southern Hemisphere simulated by a coupled atmosphere-sea ice-ocean-vegetation model, The Holocene, 15, 951-964.
- ↑ 11.0 11.1 11.2 Crosta, X., Denis, D. and Ther, O. 2008. Sea ice seasonality during the Holocene, Adelie Land, East Antarctica, Marine Micropaleontology, 66, 222-232.
- ↑ Hall, B.L., Baroni, C. and Denton, G.H. 2003. Holocene relative sea-level history of the southern Victoria Land coast, Antarctica, Global and Planetary Change, 42, 241-263.
- ↑ Belt S., Masse, G., Rowland, S.J., Poulin, M., Michel, C. and Leblanc, B. 2007. A novel chemical fossil of palaeo sea ice: IP25, Organic Geochemistry, 38, 16-27.
- ↑ Massé, G., Rowland, S., Sicre, M-A., Jacob, J., Jansen, E. and Belt, S. 2008. Abrupt climate changes for Iceland during the last millennium: Evidence from high resolution sea ice reconstructions, Earth and Planetary Science Letters, 269, 564-568.
- ↑ Iizuka, Y., Hondoh, T. and Fujii, Y. 2008. Antarctic sea ice extent during the Holocene reconstructed from inland ice core evidence, Journal of Geophysical Research, 113, D15114, doi:10.1029/2007JD009326.
- ↑ Rankin, A.M., Wolff, E.W. and Martin, S. 2002. Frost flowers: Implications for tropospheric chemistry and ice core interpretation, J. Geophys. Res., 107, 4683, doi:10.1029/2002JD002492.
- ↑ Wolff, E.W., Rankin, A.M. and Rothlisberger, R. 2003. An ice core indicator of Antarctic sea ice production?, Geophysical Research Letters, 30, 2158. (10.1029/2003GL018454.)
- ↑ Wolff, E.W., Fischer, H., Fundel, F., Ruth, U., Twarloh, B., Littot, G.C., Mulvaney, R., Röthlisberger, R., De Angelis, M., Boutron, C.F., Hansson, M., Jonsell, U., Hutterli, M.A,, Lambert, F., Kaufmann, P., Stauffer, B., Stocker, T.F., Steffensen, J.P., Bigler, M., Siggaard-Andersen, M.L., Udisti, R., Becagli, S., Castellano, E., Severi, M., Wagenbach, D., Barbante, C., Gabrielli, P. and Gaspari, V. 2006. Southern Ocean sea-ice extent, productivity and iron flux over the past eight glacial cycles, Nature, 440, 491-496 (doi:10.1038/nature04614).
- ↑ Fischer, H., Fundel, F., Ruth, U., Twarloh, B., Wegener, A., Udisti, R., Becagli, S., Castellano, E., Morganti, A., Severi, M., Wolff, E.W., Littot, G.C., Röthlisberger, R., Mulvaney, R., Hutterli, M.A., Kaufmann, P., Federer, U., Lambert, F., Bigler, M., Hansson, M., Jonsell, U., De Angelis, M., Boutron, C., Siggaard-Andersen, M.L., Steffensen, J.P., Barbante, C., Gaspari, V., Gabrielli, P. and Wagenbach, D. 2007. Reconstruction of millennial changes in dust emission, transport and regional sea ice coverage using the deep EPICA ice cores from the Atlantic and Indian Ocean sector of Antarctica, Earth and Planetary Science Letters, 260, 340-354.
- ↑ 20.0 20.1 20.2 Curran, M.A.J., Van Ommen, T.D., Morgan, V.I., Phillips, K.L. and Palmer, A.S. 2003. Ice core evidence for Antarctic sea ice decline since the 1950s, Science, 302, 1203-1206.
- ↑ Welch, K.A., Mayewski, P.A. and Whitlow, S.I. 1993. Methanesulphonic acid in coastal Antarctic snow related to sea-ice extent, Geophysical Research Letters, 20, 443-446.
- ↑ Fundel, F., Fischer, H., Weller, R., Traufetter, F., Oerter, H. and Miller, H. 2006. Influence of large-scale teleconnection patterns on methane sulfonate ice core records in Dronning Maud Land, Journal of Geophysical Research, 111, D04103.
- ↑ Abram, N.J., McConnell, J., Mulvaney, R., and Wolff, E. 2007b. Ice core records of regional sea ice changes around Antarctica during the 20th century. Geophysical Research Abstracts 01599.
- ↑ Abram, N.J., Mulvaney, R., Wolff, E. and Mudelsee, M. 2007a. Ice core records as sea ice proxies: An evaluation from the Weddell Sea region of Antarctica. Journal of Geophysical Research, 112:D15101.