Precipitation changes over the 21st century
- This page is part of the topic Atmospheric change over the next 100 years
The net precipitation (in climate models this is precipitation less evaporation and ablation) over Antarctica is an important factor in the mass balance of the continental ice sheet. Surface sublimation and blowing-snow processes also help to determine the local mass balance of the ice sheet, but these factors have a limited contribution at scales larger than 100 km (Genthon, 2004[1]) and are not included in climate models. Giovinetto et al (1997[2]) estimated the various terms and found blowing snow to contribute about 6% to the surface mass balance. Hence, quantification of precipitation changes in high southern latitudes is paramount for resolving the uncertainty surrounding the future of the Antarctic ice sheet.
Skill in simulation of precipitation
Much of the validation in model performance with regard to precipitation focuses on net precipitation, since this quantity can be calculated using a range of methodologies, and, in particular, is less dependent upon problematic and sparse station measurements of snow accumulation. In an analysis used in the AR4, Uotila et al. (2007[3]) found that only 5 of 15 global climate models examined were able to simulate long term average values of net precipitation consistent with the range of observations in the Twentieth Century. This range falls approximately between 150 and 190 mm/year, although recent analyses suggest the likely figure is at the high end of this range (Monaghan et al. (2006a[4]) quoted 182 mm/year). Monaghan et al. (2006a[4]) combined new records from the International Transantarctic Scientific Expedition (ITASE) with existing ice cores, snow pit and snow stake data, meteorological observations, and validated model fields to reconstruct Antarctic snowfall accumulation over the past 5 decades, and concluded that there had been no trend over that time, although a significant increase in the net precipitation has been reported in the immediate area surrounding the South Pole, based on in situ measurements (Mosley-Thompson et al., 1999[5]) and on satellite altimetry (Davis et al., 2005[6]). It is worth noting that this South Pole area is very small in comparison with the area studied by Monaghan et al. (2006a[4]), and may well have been affected by the existence of the station there (a significant snow hill is also associated with Byrd’s former station on the Ross Ice Shelf).
Observational uncertainty, including uncertainties in trend detection, means that an assessment of simulation skill remains particularly difficult. Nevertheless, it is known that specific deficiencies remain in the parameterizations of key processes that drive precipitation. This is particularly true of the polar regions, since polar cloud microphysics remains poorly understood. The importance of the moisture physics is further demonstrated by studies such as that by Turner et al. (2006[7]), who found that local thermodynamic processes were a significant component of the climate change signal in the Antarctic winter. In the context of the SHEBA (Surface Heat Budget of the Arctic – an experiment carried out in the Beaufort and Chukchi Sea region of the Arctic in 1997-1998) experiment in the Arctic, much work has been done on the development of polar cloud physics parameterizations, but significant challenges remain even in fine scale models (Sandvik et al., 2007[8]). In the Antarctic, there has been less focused development of the physical parameterizations needed to better represent clouds and precipitation, largely due to the absence of an intense field programme to provide the necessary data support for such an endeavour. The precipitation simulations in high southern latitudes by global models result in significant biases (e.g. Covey et al., 2003[9]), and this is also true, though not as severe, in high resolution, limited area models (e.g. Bromwich et al., 2004a[10]; Van de Berg et al., 2005[11]). Hines et al. (2004[12]) found that in one global model, the simulation of Antarctic climate was highly sensitive to the mixing ratio threshold for autoconversion from suspended ice cloud to falling precipitation. Such sensitivity can only be resolved by measurements that are currently not available.
An important driving mechanism for precipitation in this region is the atmospheric circulation (e.g. Massom et al., 2004[13]). The contributions of the multi-year and the synoptic time scales are roughly proportional over the coastal regions, but the synoptic time scale dominates the inland precipitation (Cullather et al., 1998[14]). This component exhibits a close relationship with elevation and makes a positive contribution to transporting moisture from the ocean toward the pole. Global models exhibit significant biases in this regard also. Placed in the context of the available re-analyses, the multi-model ensemble created for the AR4 overestimates pressures over the ice sheet in summer and overestimates cyclone depths, particularly in the west Antarctic region, in all seasons (Lynch et al., 2006[15]). Two issues of particular note in driving these deficiencies are surface forcing and spatial scale. With regard to the former, Stratton and Pope (2004[16]) have noted that Arctic Model Intercomparison Project-style experiments (that is, model simulations with specified sea surface temperatures) do produce correctly located storm tracks, but often even these are more zonally oriented than is observed. Krinner et al. (2007[17]) have found that errors in net precipitation on regional scales are moderated when observed sea surface conditions are prescribed. With regard to the latter, Bromwich et al. (2004b[18]) noted that the reanalysis products, and therefore probably global models in general, underestimate Antarctic precipitation in the Twentieth Century. This is attributed to the smooth coastal escarpment in a coarse resolution model, which causes cyclones to precipitate less than they do in reality. Further, if the Antarctic Peninsula is not well resolved in a model, it produces too little lee cyclogenesis (Turner et al., 1998[19]).
Projected changes in precipitation
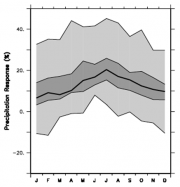
The lack of a Twentieth Century trend in net precipitation in recent comprehensive analyses is particularly problematic in the context of Twenty First Century model projections. Almost all climate models simulate a continuing robust precipitation increase over Antarctica in the coming century (see Figure 5.9) The projected precipitation change has a seasonal dependency, and is larger in winter than in summer. In some models, there is also a phase shift, so that, for example, a narrow early winter peak in precipitation evolves to a broad winter peak (Krinner et al., 2007[17]). Wild et al. (2003[20]) reported that, in a simulation in which the CO2 concentration in the atmosphere doubles, the annual net accumulation over Antarctica increases by 22 mm/year. Similarly, Huybrechts et al. (2004[21]) analyzed results from an ice sheet model driven by a climate model simulation in which the CO2 concentration doubles in 60 years, and found an associated increase of 15% to 20% in mean Antarctic precipitation. The increasing net precipitation in most climate models reflects warmer air temperatures and associated higher atmospheric moisture. Evaporation increases also, but does not keep pace with the precipitation increases. Two models, ECHO-G and GFDL-2.1, project a small decrease of net precipitation after the middle of the Twenty First Century. Interestingly, these models fall at either end of the quality scale in assessments of their ability to reproduce Antarctic synoptic climate, according to Uotila et al. (2007[3]).
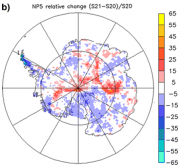
Only one study has attempted to address the projected changes in the intensity of precipitation. Krinner et al. (2007[17]) calculated the difference in the number of days per year with daily precipitation exceeding five times the mean daily precipitation for the simulations using the LMDZ4 stretched grid atmospheric model (see Figure 5.10) This particular model experiment suggests that even using this relatively modest measure, which does not allow for the increase in total precipitation, the number of relatively strong precipitation events near the ice sheet domes and ridges increases, particularly in East Antarctica. This indicates an increased frequency of intrusions of moist marine air, in spite of a projected lower future cyclone frequency.
A separate, and biologically significant, requirement for inclusion in prediction of future precipitation patterns is the ability to differentiate between precipitation as snow and as rain. While this is not relevant across most of East Antarctica, it is a change that is already apparent along the western Antarctic Peninsula and Scotia arc archipelagos. Precipitation falling as rain is immediately available to terrestrial biota, and hence has some more direct impacts on terrestrial ecosystem changes than that falling as snow.
The scatter among the individual models reported on in the AR4 is considerable. During the first half of the Twenty First Century, models using the A1B scenario predict anything from a maximum upward trend of 0.71 mm/year to a maximum downward trend of 0.13 mm/year. Some models project stronger net precipitation increases in the first half of the Twenty First Century, while other models project stronger increases after the middle of the century. Hence, the confidence in any conclusions arising from AR4 or this report regarding the future trajectory of Antarctic precipitation is extremely limited.
The study of Bracegirdle et al. (2008[22]) estimated that by the end of the century the snowfall rate over the continent would increase by 20% compared to current values, which, if other effects such as melting and dynamical discharge are ignored, would result in a negative contribution to global sea-level rise of approximately 5 cm.
References
- ↑ Genthon, C., 2004: Space-time Antarctic surface mass-balance variability from climate models, Ann. Glaciol., 39, 271-275.
- ↑ Giovinetto, M.B., Yamazaki, K., Wendler, G. and Bromwich, D.H. 1997. Atmospheric net transport of water vapor and latent heat across 60 degrees S, J. Geophys. Res., 102, 11171-11179.
- ↑ 3.0 3.1 Uotila P., Lynch, A.H., Cassano, J.J. and Cullather, R.I. 2007. Changes in Antarctic net precipitation in the 21st century based on Intergovernmental Panel on Climate Change (IPCC) model scenarios, J. Geophys. Res., 112, D10107, doi:10.1029/2006JD007482.
- ↑ 4.0 4.1 4.2 Monaghan, A.J., Bromwich, D.H. and Wang, S-H. 2006a. Recent trends in Antarctic snow accumulation from Polar MM5, Philosophical Trans. Royal. Soc. A, 364, 1683-1708.
- ↑ Mosley-Thompson, E., J. F. Paskievitch, A. J. Gow, L. G. Thompson, 1999: Late 20th century increase in South Pole snow accumulation, J. Geophys. Res., 104(D4), 3877-3886, 10.1029/1998JD200092.
- ↑ Davis, C.H., Li Y., McConnell, J.R., Frey, M.M. and Hanna, E. 2005. Snowfall-driven growth in East Antarctic Ice Sheet mitigates recent sea-level rise, Science, 308, 1898-1901.
- ↑ Turner, J., Lachlan-Cope, T.A., Colwell, S.R., Marshall, G.J. and Connolley, W.M. 2006. Significant warming of the Antarctic winter troposphere, Science, 311, 1914-1917.
- ↑ Sandvik, A., Biryulina, M., Kvamsto, N.G., Stamnes, J.J. and Stamnes, K. 2007. Observed and simulated microphysical composition of arctic clouds: Data properties and model validation, J. Geophys. Res., 112, Art. No. D05205.
- ↑ Covey, C., Achutarao, K.M., Cubasch, U., Jones, P., Lambert, S.J., Mann, M.E., Phillips, T.J. and Taylor, K.E. 2003. An overview of results from the Coupled Model Intercomparison Project (CMIP), Global Planet. Change, 37, 103-133, doi:10.1016/S0921-8181(02)00193-5.
- ↑ Bromwich, D.H., Guo, Z., Bai, L. and Chen, Q-S. 2004a. Modeled Antarctic precipitation. Part I: spatial and temporal variability, J. Climate, 17, 427-447.
- ↑ Van De Berg, W.J., Van Den Broeke, M.R., Reijmer, C.H. and Van Meijgaard, E. 2005. Characteristics of the Antarctic surface mass balance (1958-2002) using a regional atmospheric climate model, Ann. Glaciol., 41, 97-104.
- ↑ Hines, K.M., Bromwich, D.H., Rasch, P.J. and Iacono, M.J. 2004. Antarctic Clouds and Radiation within the NCAR Climate Models, J. Climate, 17, 1198-1212.
- ↑ Massom, R.A., Pook, M.J., Comiso J.C., Adams, N., Turner, J., Lachlan-Cope, T. and Gibson, T.T. 2004. Precipitation over the interior East Antarctic Ice Sheet related to midlatitude blocking-high activity, J. Climate, 17, 1914-1928.
- ↑ Cullather, R.I., Bromwich, D.H. and Van Woert, M.L. 1998. Spatial and temporal variability of Antarctic precipitation from atmospheric methods, J. Climate, 11, 334-367.
- ↑ Lynch, A., Uotila, P. and Cassano, J.J. 2006. Changes in synoptic weather patterns in the polar regions in the twentieth and twenty-first centuries, Part 2: Antarctic, Int. J. Climatol., 26, 1181-1199.
- ↑ Stratton, R.A. and Pope, V.D. 2004. Modelling the climatology of storm tracks - Sensitivity to resolution. In: The Second Phase of the Atmospheric Model Intercomparison Project (AMIP2) [Gleckler, P. (ed.)]. Proceedings of the WCRP/WGNE Workshop, Toulouse, 207-210.
- ↑ 17.0 17.1 17.2 17.3 Krinner, G., Magand, O., Simmonds, I., Genthon, C. and Dufresne J.L. 2007. Simulated Antarctic precipitation and surface mass balance at the end of the twentieth and twenty-first centuries, Clim. Dyn., 28, 215-230.
- ↑ Bromwich, D.H., Monaghan, A.J. and Guo, Z. 2004b. Modeling the ENSO modulation of Antarctic climate in the late 1990s with the Polar MM5, J. Clim., 17, 109-132.
- ↑ Turner, J., Leonard, S., Lachlan-Cope, T., and Marshall, G.J. 1998. Understanding Antarctic Peninsula precipitation distribution and variability using a numerical weather prediction model, Ann. Glaciol., 27, 591-596.
- ↑ Wild, M., Calanca, P., Scherrer, S.C. and Ohmura, A. 2003. Effects of polar ice sheets on global sea level in high-resolution greenhouse scenarios, J. Geophys. Res., 108, 4165, doi:10.1029/2002JD002451.
- ↑ Huybrechts, P., Gregory, J., Janssens, I. and Wild, M. 2004. Modelling Antarctic and Greenland volume changes during the 20th and 21st centuries forced by GCM time slice integrations, Glob. Planet. Change, 42, 83-105.
- ↑ Bracegirdle, T.J., Connolley, W.M. and Turner, J. 2008. Antarctic climate change over the Twenty First Century, Journal of Geophysical Research – Atmospheres, 113, D03103, doi:03110.01029/02007JD008933.