Impacts of instrumental period climate change on macroalgae
- This page is part of the topic Marine biology in the instrumental period
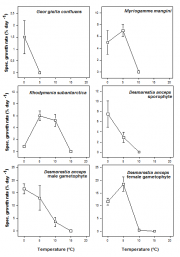
Many Antarctic macroalgae, especially from the subtidal zone, are adapted to low temperatures (Wiencke et al., 1994[2], 2007[3]), especially those endemic to the Antarctic. For example, the red algae Georgiella confluens (Figure 4.53), Gigartina skottsbergii and Plocamium cartilagineum grow only at 0°C and not at 5°C, and exhibit upper survival temperatures (USTs) as low as 7 to 13-14°C, respectively (Bischoff-Bäsmann and Wiencke, 1996[4]). Other Antarctic red algae grow at temperatures up to 5 or 10°C and have USTs of up to 19°C. Macroscopic growth stages of endemic Antarctic brown algae grow up to 5°C and exhibit USTs of 11 to 13°C. Their reproductive microscopic stages grow up to 10 or 15°C and have USTs between 15 and 18°C (Wiencke and tom Dieck, 1989). Antarctic cold-temperate species (especially from the intertidal) are characterised by higher temperature requirements (Wiencke and tom Dieck, 1990).
This adaptation of Antarctic macroalgae to low temperatures is also reflected in photosynthesis. The photosynthetic capacities of endemic Antarctic species measured at 0°C are as high as in temperate algae measured at higher temperatures (Thomas and Wiencke, 1991[5]; Wiencke et al., 1993[6]; Weykam et al., 1996[7]; Eggert and Wiencke, 2000[8]). However, optimum temperatures for photosynthesis are clearly above the Antarctic water temperatures. The lowest temperature optima have been recorded in Ascoseira mirabilis (1 to 10°C). The other species tested so far are characterised by higher values between 10 and 20°C. Temperature optima for dark respiration are higher than those for photosynthesis (Drew, 1977[9]; Wiencke et al., 1993[6]; Eggert and Wiencke, 2000[8]).
Due to global warming the geographic distribution of stenothermal cold water species will be limited in future to the coasts of the Antarctic continent itself. Species presently occurring today predominantly in the cold-temperate region and rarely in the Antarctic will become more established in the Antarctic region (Müller et al., submitted). This can cause unforeseeable changes in the ecology of Antarctic shallow water communities.
Light determines significantly the depth zonation and survival of macroalgae. Antarctic seaweeds are able to tolerate dark periods of up to one year without suffering damage, and their light requirements for growth are very low (Wiencke, 1990[10]). In juvenile sporophytes of Antarctic Desmarestiales, growth is light saturated at ≤ 20 µmol photons/m2/s1 (Wiencke and Fischer, 1990[11]). The low light requirements for completion of the life cycle and for growth of Antarctic seaweeds are based on the low light requirements for photosynthesis (Wiencke et al., 1993[6]; Weykam et al., 1996[7]; Eggert and Wiencke, 2000[8]). As a result, light compensation (Ic) and saturation (Ik) points are very low in Antarctic species and mostly range between 1 and 15 and between 14 and 52 µmol photons/m2/s1, respectively (Wiencke et al., 1993[6]; Weykam et al., 1996[7]; Brouwer, 1996[12]; Eggert and Wiencke, 2000[8]). These low light requirements allow Antarctic macroalgae to grow down to considerable depths. Theoretically, growth of some species is possible at water depths down to approximately 75 m (Wiencke, 1990[10]; Wiencke et al., 1993[6]).
Antarctic algae are not only low light adapted but can also cope with high light conditions in summer due to their ability for dynamic photoinhibition, a photoprotective mechanism by which excessive energy absorbed is rendered harmless by thermal dissipation. The capability for dynamic photoinhibition is related to the depth distribution of the individual species. Species from the eulittoral, upper and mid sublittoral show a more or less pronounced decrease in photosynthetic activity during high light stress, and full recovery during subsequent exposure to dim light. In contrast, deep water and understory species recover only slightly and slowly indicating photodamage, especially after exposure to UV radiation. Recent studies indicate that the effect of UV radiation is not limited to the physiological and organism level. Rather, it influences the biodiversity, structure and function of macroalgal communities (Zacher et al., 2007[13]; Campana et al., submitted). The effects on the community level depend on the UV susceptibility of the unicellular propagation units of the seaweeds (Roleda et al., In Press; Zacher et al., 2009[14]). So UV radiation represents an important factor for the determination of the upper distribution limit on the shore. The obtained results are of great importance for the estimation of the effects of enhanced UV radiation due to stratospheric ozone depletion. UV induced changes in algal zonation and biodiversity make changes in the trophic relations of coastal ecosystems very probable.
References
- ↑ Wiencke, C. and Clayton, M.N. 2002. Antarctic Seaweeds, (Synopses of the Antarctic Benthos. Ed.: J. W. Wägele and J. Sieg ; Vol. 9), Ruggell : Gantner ; Königstein : Koeltz Scientific Books., 239 pp.
- ↑ Wiencke, C., Bartsch, I., Bischoff, B., Peters, A.F. and Breeman, A.M. 1994. Temperature requirements and biogeography of Antarctic, Arctic and amphiequatorial seaweeds, Botanica Marina., 37, 247-259.
- ↑ Wiencke, C., Clayton, M. N., Gómez, I., Iken, K., Lüder, U. H., Amsler, C. D., Karsten, U., Hanelt, D., Bischof, K. and Dunton, K. 2007. Life strategy, ecophysiology and ecology of seaweeds in polar waters, Reviews in Environmental Science and Biotechnology, 6(1/3), 95-126.
- ↑ Bischoff-Bäsmann, N. and Wiencke, C. 1996. Temperature requirements for growth and survival of Antarctic Rhodophyta, Journal of Phycology, 32, 525-535.
- ↑ Thomas, D.N. and Wiencke, C. 1991. Photosynthesis, dark respiration and light independent carbon fixation of endemic Antarctic macroalgae, Polar Biol., 11, 329-337.
- ↑ 6.0 6.1 6.2 6.3 6.4 Wiencke, C., Rahmel, J., Karsten, U., Weykam, G. and Kirst, G.O. 1993. Photosynthesis of marine macroalgae from Antarctica Light and temperature requirements, Botanica Acta, 106, 78-87.
- ↑ 7.0 7.1 7.2 Weykam, G., Gómez, I., Wiencke, C., Iken, K. and Klöser, H. 1996. Photosynthetic characteristics and C:N ratios of macroalgae from King George Island (Antarctica), Journal of Experimental Marine Biology and Ecology, 204, 1-22.
- ↑ 8.0 8.1 8.2 8.3 Eggert, A., Wiencke, C. 2000. Adaptation and acclimation of growth and photosynthesis of five Antarctic red algae to low temperatures, Polar Biol., 23, 609-618.
- ↑ Drew, E.A. 1977. The physiology of photosynthesis and respiration in some Antarctic marine algae, Br. Antarct. Surv. Bull., 46, 59-76.
- ↑ 10.0 10.1 Wiencke, C. 1990. Seasonality of brown macroalgae from Antarctica - a long-term culture study under fluctuating Antarctic daylengths, Polar Biol., 10, 589-600.
- ↑ Wiencke, C. and Fischer, G. 1990. Growth and stable carbon isotope composition of cold-water macroalgae in relation to light and temperature, Mar. Ecol. Prog. Ser., 65, 283-292.
- ↑ Brouwer, P.E.M. 1996. In situ photosynthesis and estimated annual production of the red macroalga Myriogramme mangini in relation to underwater irradiance at Signy Island (Antarctica), Antarct. Sci., 8, 245-252.
- ↑ Zacher, K., Wulff, A., Molis, M., Hanelt, D. and Wiencke, C. 2007. Ultraviolet radiation and consumer effects on a field-grown intertidal macroalgal assemblage in Antarctica, Global Change Biology, 13(6), 1201-1215.
- ↑ Zacher, K., Roleda, M.Y., Wulff, A., Hanelt, D., and Wiencke, C. 2009. Responses of Antarctic Iridaea cordata (Rhodophyta) tetraspores exposed to ultraviolet radiation. Phycological research, 57(3), 186-193.