Biological modelling and bioregionalization
- This page is part of the topic Models of the physical and biological environment of the Antarctic
In the Antarctic environmental conditions, particularly on land but also in the sea can show high variability at both, spatial and temporal scales. This is especially relevant in the context of climate change, because the area of intensive atmospheric and sea water surface warming west of the Antarctic Peninsula coincides largely with the off-shore area that is central to large krill swarms, their population dynamics and consequently, higher trophic levels of the open ocean ecosystem. Further, inshore benthic communities range regionally from those with the world record biomass values within filter feeding assemblages (Gutt, 2000[1]) to others with possibly the lowest community metabolic rates in the extremely depauperate species poor benthic systems that thrive below ice shelves (Azam et al., 1979[2]). Temporal variations can be very important, e.g. in krill and penguin populations, benthic recruitment, and primary production, and are either supported or reduced by the occurrence of sea ice (Beaman and Harris, 2005[3]; Gutt et al., 2007[4]).
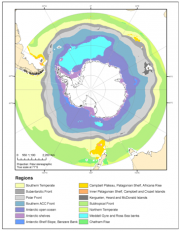
As a consequence of these constraints, advanced ecosystem research, independently of whether it refers to singe species or to communities, to ecosystem functioning or complex structures, demands spatially and temporarily explicit models. The approach of comparing various ecological layers (i.e. parameters) to understand the functioning of the ecosystem, including its spatial variability, is known as “bioregionalization” (Figure 2.36), and has the aim of identifying ecological “hot-” and “cold-spots”. This concept can also be used to answer specific ecological questions - e.g. to understand the habitat of an Antarctic midwater fish and its trophic position in the pelagic ecosystem, with the objective of predicting fish recruitment (Loots et al., 2007[6]).
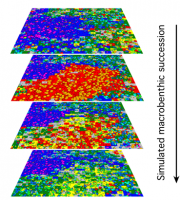
In cases where a complete coverage of important ecological parameters and organisms, even within a limited area is not possible (e.g. for bottom communities), a series of proxies such as water depth, bathymetry, water masses and currents as well as ecological key species can be used to estimate benthic structures and processes. Later, the potential for circumpolar application can be tested. For a general understanding of selected processes even individual based biodiversity-models can elucidate the relationship between, for example, climate-induced iceberg disturbance in combination with biological characteristics, such as dispersal, reproduction, mortality, and longevity of model organisms (Johst et al., 2006[7]; Potthoff et al., 2006[8]). Such models can cover periods of faunistic succession which exceed by orders of magnitudes the life span of ordinary scientific projects, and simulate expected climate change scenarios, e.g. the response of the benthos to the absence of iceberg scouring or to extremely high magnitudes of disturbance (Figure 2.37).
In addition spatially explicit biological models can contribute to solve methodological problems such as autocorrelation and can help to maximize the efficiency of sampling designs.
With advanced studies of ecosystem research uni-dimensional parameters can either be relatively easily sampled using satellite data (e.g. in the case of ice cover and sea surface temperature), or demand high effort field studies (e.g. in the case of krill or fish stocks). Most multidimensional biological approaches will never be able to cover the entire area of the Southern Ocean and a complete range of organisms. To study parameters, which explain biodiversity, ecosystem functioning (such as reproduction, dispersal, feeding, or physiological tolerance), and climate sensitivity ecological key specied can be selected.
In the next step of advanced bioregionalization research, some of the approaches described above could be merged into one model with the option of working at different levels of resolution, spatially, temporarily, physically and biologically. Finally, such knowledge can provide the best possible basis to identify “Vulnerable Marine Ecosystems” (VME), options for Marine Protected Areas (MPA) and produce numerical predictions for the future of the Antarctic ecosystem. This has recently been identified by SCAR as an important challenge, resulting in the formation of an action group on “Prediction of Changes in the Physical and Biological Environment of the Antarctic”.
Based on such knowledge the UN Environmental Programme, World Conservation Monitoring Centre (UNERP-WCMC) developed in cooperation with the Conference on Biological Diversity (CBD), other international institutions and specialists criteria to identify “Ecologically and biologically significant areas“ (EBASs) to propose these as “Marine Protected Areas” (MPA’s). In parallel the Convention on the Conservation of Antarctic Marine Living Ressources (CCAMLR) has started to declare “Vulnerable Marine Ecosystems” (VME) to protect them from anthropogenical impact, mainly ground fishing.
References
- ↑ Gutt, J. 2000. Some “driving forces” structuring communities of the sublittoral Antarctic macrobenthos, Antarctic Sci., 12 (3), 297-313.
- ↑ Azam, F., Beers, J.R., Campbell, L., Carlucci, A.F., Holm-Hansen, O., Reis, F.M.H. and Karl, D.M. 1979. Occurrence and metabolic activity of organisms under the Ross Ice Shelf, Antarctica, at station J9, Science, 203, 451-453.
- ↑ Beaman, R.J. and Harris, P.T. 2005. Bioregionalization of the George V Shelf, East Antarctica, Cont. Shelf Res., 25, 1657-1691.
- ↑ Gutt, J., Koubbi, P. and Eléaume, M. 2007. Mega-epibenthic diversity off Terre Adélie (Antarctica) in relation to disturbance, Polar Biol., 30, 1323-1329.
- ↑ Grant, S., Constable, A., Raymond, B. and Doust, S. 2006. Bioregionalisation of the Southern Ocean, Report of Experts Workshop, Hobart, September 2006. WWF-Australia and ACE CRC.
- ↑ Loots, C., Koubbi, P. and Duhamel, G. 2007. Habitat modelling of Electrona antarctica (Myctophidae, Pisces) in Kerguelen by generalized additive models and geographic information systems, Polar Biol., 30, 951-959.
- ↑ Johst, K., Gutt, J., Wissel, C. and Grimm, V. 2006. Diversity and disturbances in the Antarctic megabenthos: feasible versus theoretical disturbance ranges, Ecosystems, 9, 1145-1155.
- ↑ Potthoff, M., Johst, K. and Gutt, J. 2006. How to survive as a pioneer species in the Antarctic benthos: minimum dispersal distance as a function of lifetime and disturbance, Polar Biol., 29, 543-551.